Unhealthy diets and sedentary lifestyle have led to the development of overweight and obesity and have largely contributed to the current epidemic of chronic diseases, especially CVD and type 2 diabetes which both represent a significant burden to society and a very high cost to the global economy(Reference Hruby and Hu1). One well-acknowledged issue is that these pathologies can be partially prevented and reduced by targeting a number of modifiable lifestyle risk factors(Reference Rao2). Specific changes in dietary patterns constitute one of the most effective and advisable preventive strategies insofar as healthier diets could prevent one in five deaths globally(Reference Collaborators3). Modification of the dietary habits can have an impact on a range of cardiometabolic factors (e.g. serum lipids, blood pressure, endothelial function and glucose–insulin homeostasis), can influence specific body defence systems (e.g. anti-inflammatory and antioxidant status) and also affect the gut microbiome. While there is no single optimal diet for cardiometabolic health, consistent evidence has emerged showing that beneficial health outcomes are associated with high adherence to the Mediterranean, DASH (dietary approaches to stop hypertension) or healthy Nordic diets(Reference Reedy, Krebs-Smith and Miller4–Reference Uusitupa, Hermansen and Savolainen6). All these diets emphasise the intake of plant foods, including fruit, vegetables, nuts, whole grains and cereals, which in addition to their low energy and salt supply, low glycaemic index and richness in essential micronutrients and fibres also provide a high number and variety of bioactive compounds (also called phytochemical) to which a range of biological properties and beneficial effects have been attributed(Reference Mozaffarian7).
Phytochemicals are secondary metabolites produced by plants in response to pathogens or abiotic stress. They are not toxic to human subjects as long as they are administered in dietary doses. Depending on their basic chemical structure, they are classified into different families, some of which are quite ubiquitous whereas others are specifically present only in some foods. The most abundant families of phytochemicals in our diet are polyphenols (including flavonoid and non-flavonoid compounds such as phenolic acids, lignans, ellagitannins, curcuminoids and stilbenes), carotenoids (carotenes and xanthophylls), alkaloids, plant sterols (phyto-sterols and phyto-stanols), sulphur-containing compounds and glucosinolates (Fig. 1). Their respective levels of intake can differ substantially depending on dietary habits and food preferences. Nevertheless, the daily intake of food phytochemicals far exceeds that of vitamins and often reaches >1 g/d(Reference Zamora-Ros, Knaze and Rothwell8).
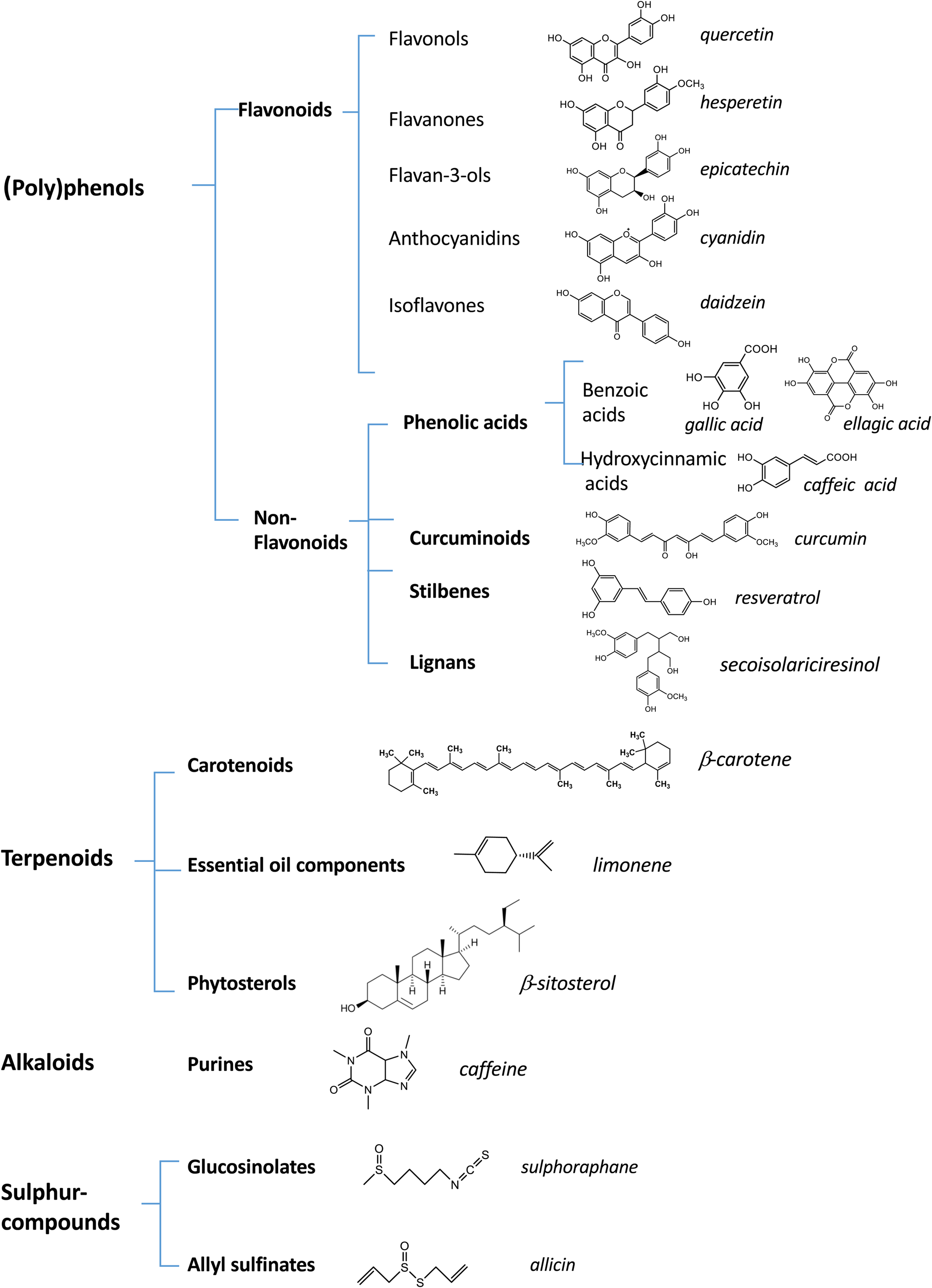
Fig. 1. (Colour online) Diversity of bioactive compounds in plant foods. This figure shows the most important categories of dietary phytochemicals and their distribution into different classes and subclasses. The chemical structures and the names (in italics) of some representative compounds for each class or subclass are presented.
Many phytochemicals, especially polyphenols and plant sterols, have long been investigated in in vitro and animal studies, which have provided pre-clinical evidence of a variety of biological effects that are known to be involved in the regulation and maintenance of cardiometabolic health(Reference Cicero, Fogacci and Colletti9). For example, some polyphenols, as well as some of their in vivo metabolites, have been shown to modulate the activity of enzymes involved in carbohydrate digestion, redox processes, the production and bioactivity of nitric oxide and the blood pressure regulatory mechanisms among others(Reference Cicero, Fogacci and Colletti9–Reference Hanhineva, Torronen and Bondia-Pons11). The molecular regulations potentially underlying these effects have also been long studied. It is postulated that modulation of gene expression, via transcriptional and (or) epigenetic changes, as well as the interference with key signalling metabolic regulatory pathways (e.g. glucose–insulin homeostasis, dyslipidaemia, energy metabolism, inflammation, redox homeostasis, vascular tone and integrity), may be involved in the metabolic benefits of the intake of phytochemicals(Reference Kerimi and Williamson12–Reference Upadhyay and Dixit14). These biological and molecular responses might be partially mediated by the interaction of the polyphenolic compounds and metabolites with the host cells and tissues, an issue that is controlled by a limited and variable systemic availability of the phytochemicals (e.g. plasma and host tissue levels are generally in the nm to μm range)(Reference Del Rio, Rodriguez-Mateos and Spencer15,Reference Manach, Williamson and Morand16 ). Importantly, a large proportion of these plant food bioactives ingested reach the colon intact where they are able to promote the growth of beneficial bacteria (prebiotic activity) with potential cardiometabolic benefits(Reference Anhe, Roy and Pilon17,Reference Duenas, Munoz-Gonzalez and Cueva18 ).
In human subjects, a growing number of randomised controlled trials (RCT) and meta-analysis have provided evidence of the improvement of a range of biomarkers of cardiometabolic risk in response to the intake of specific phytochemical-rich or enriched foods and/or isolated food phytochemicals(Reference Del Bo, Bernardi and Marino19–Reference Demonty, Ras and van der Knaap23). However, the true contribution of these plant bioactives to the health benefits associated with the consumption of their food sources might have been underestimated, mainly due to the marked differences in the responses observed between individuals enrolled in the same clinical trial, leading to mixed results of the intervention(Reference Manach, Milenkovic and Van de Wiele24,Reference Jones25 ). Such heterogeneity can affect the magnitude of the average change detected in a sample population for a specific biomarker as well as the statistical and biological significance of that change(Reference Morand and Tomas-Barberan26). The observed heterogeneity suggests that there may be subgroups of individuals with a larger and (or) more significant response than others and that the bio-efficacy of food phytochemicals to improve cardiometabolic health could be population specific.
An important issue is now to establish which intrinsic and extrinsic factors are responsible for the interindividual variation for the main families of plant food bioactives. As recently reported, several factors, i.e. genetic background, gut microbiota composition and functionality (microbiome), health status, age or sex, may explain interindividual variations for at least some of the phytochemicals(Reference Manach, Milenkovic and Van de Wiele24,Reference Milenkovic, Morand and Cassidy27 ). However, this research area is poorly investigated. To review the current limited and fragmented knowledge and identify the main gaps in knowledge, the European scientific network, European Cooperation in Science and Technology (COST)-plant food bioactives interindividual variation (POSITIVe), gathered experts from different disciplines, including nutrition and plant food bioactives, food science, clinical research, microbiology, gut microbiome, genetics, nutrigenomics, bioinformatics and molecular biology, to tackle, using a multidimensional approach, the complex question of the interindividual variation in response to the consumption of the major families of plant food bioactives. This network has combined and analysed, in a systematic way, the available knowledge with the following key scientific objectives: (1) to identify the key factors that modulate individual variation of the bioavailability (absorption, distribution, metabolism and excretion (ADME)) for the main families of plant food bioactives, as well as to improve methods and tools to assess individual exposure; (2) to identify, beyond bioavailability, the main factors that are responsible for between-subject variation in the biological response for cardiometabolic endpoints, and to assess the variability in the nutrigenomic response to the intake of plant food bioactives; and (3) to integrate findings and to set out the gaps and needs for future research.
This review presents the main findings resulting from the COST-POSITIVe network activities and also the recommendations for future research to allow a better understanding and the prediction of the individual responses to the consumption of plant food bioactives.
Interindividual variation in the bioavailability of plant food bioactives
To determine the main factors involved in the between-subjects variability in the ADME of the major families of plant food bioactives, the COST-POSITIVe network has undertaken an extensive literature review (>3000 articles) on the variability of the ADME in human subjects for several classes of compounds (e.g. carotenoids, flavonoids, lignans, phenolic acids and ellagitannins), thereby covering factors such as genetic background, gut microbiota, sex and age. Despite the fact that bioavailability of many compounds is rather well documented, very few studies have been performed to identify the determinants of interindividual variability(Reference Gibney, Milenkovic and Combet28,Reference Landberg, Manach and Kerckhof29 ). This means that whilst interindividual variability has been consistently observed, the reasons or causes of the interindividual differences are far from being understood.
Polymorphisms in genes involved in plant food bioactive metabolism, from absorption to elimination, may constitute an important determinant of their bioavailability. In this regard, the available literature on carotenoids suggests that between-subject differences in the genetic background highly impact their ADME. For example, SNP in a variety of genes coding for proteins governing the micellisation process, the intestinal uptake and efflux, the metabolism and the intracellular transport have been extensively identified as key determinants of the carotenoid status, especially regarding lutein, lycopene or β-carotene(Reference Bohn, Desmarchelier and Dragsted30,Reference Bohn, Desmarchelier and El31 ). By contrast, for phenolic compounds, there is limited evidence of the influence of genetic variations on interindividual variability, probably because key information on the transporters and metabolising enzymes involved in their ADME is still missing. So far, using a candidate gene approach focusing on genes encoding polyphenols phase I and II biotransformation enzymes, researchers have only identified one polymorphism in catechol-O-methyl transferase which reduces the enzyme activity as well as several isoforms of uridine 5′diphosphoglucuronosyltransferase genes which can affect their metabolism and elimination(Reference Inoue-Choi, Yuan and Yang32,Reference Lampe33 ). Thus, the potential contribution of such genetic variations to the in vivo circulating concentrations and/or bioefficacy of polyphenols remain to be established. Based on the analysis of the current state of knowledge, the COST-POSITIVe network proposes a strategy to identify the variants in genes involved in plant bioactive metabolism, together with variants in adjacent regulatory regions, which can also influence the gene expression(Reference Landberg, Manach and Kerckhof29). The objective of this approach is to establish a list of SNP, which could be used to study genotype×ADME×phenotype associations in future clinical trials and cohort studies.
Most polyphenols pass through the small intestine without being absorbed, thus encountering the gut microbiota, which colonises the colon(Reference Scalbert and Williamson34). The gut microbiome plays a key role in the metabolism and absorption of a range of (poly)phenolic compounds, mediated through an array of metabolic capacities that can transform the native compounds into a diversity of smaller metabolites. Flavonoids and phenolic acids are most often present in foods as glycosides and esterified forms, respectively. The gut microbiota is essential for the breakdown of these glycosides and ester bonds, resulting in the release of the aglycone forms that can then be absorbed and further conjugated by phase II liver enzymes. Variations in the levels of gut microbial rhamnosidase and esterase activities could explain part of the observed interindividual variability in flavanone and hydroxycinnamic acid absorption; however, no correlation between their ADME and the gut microbiota composition has been established so far(Reference Bento-Silva, Koistinen and Mena35,Reference Tomas-Navarro, Vallejo and Sentandreu36 ). For some categories of polyphenols, the gut microbial metabolism has also been consistently demonstrated to produce highly bioactive metabolites that are also better absorbed than their native compounds(Reference Tomas-Barberan, Selma and Espin37). However, the capacity to produce gut microbial metabolites is highly variable between individuals, with polyphenol ‘metabotypes’ described for soya isoflavones, ellagic acids and ellagitannins from berries and nuts as well as for isoxanthohumol from hop(Reference Bolca, Possemiers and Maervoet38–Reference Tomas-Barberan, Garcia-Villalba and Gonzalez-Sarrias40). For example, depending on the capacity of the gut microbiota to convert ellagitannins into urolithins, corresponding to microbial-derived molecules that have been shown to affect some biomarkers of cardiometabolic risk positively, three different metabotypes have been identified in human subjects (A, B, 0)(Reference Tomas-Barberan, Gonzalez-Sarrias and Garcia-Villalba41). So far, improvement in risk biomarkers has been only reported with metabotype B(Reference Gonzalez-Sarrias, Garcia-Villalba and Romo-Vaquero42).
The information available on the specific gut bacterial strains that are able to convert these polyphenols into bioactive metabolites is currently incomplete and fragmented(Reference Braune and Blaut43). To better understand the links between differences in gut microbiota composition and the individual response to food polyphenols intake, the COST-POSITIVe network has used a strategy called gene-centric microbial genome and metagenome mining(Reference Prakash and Taylor44). The aim was to map the microbial genes/gene cassettes of interest encoding for specific and unique metabolising enzymes and transport proteins, identifying those that may differ between individuals depending on their gut microbiome(Reference Landberg, Manach and Kerckhof29). The stratification of human subjects according to their microbial metagenomic profile is hampered by difficulties notably due to a lack of accurate gene annotation and database implementation. In order to progress in this field, it will be important to acquire more robust and comprehensive information on the metabolic pathways for many compounds.
The extensive literature surveys as part of COST-POSITIVe have not necessarily led to enhancing our understanding of the role of age, sex or pathophysiological status in the interindividual variation in the ADME of the different categories of plant food bioactives. This is mostly because appropriate data to perform this analysis were not available, as observed for example for flavonols(Reference Almeida, Borge and Piskula45). Nevertheless, after gut microbiota, age and sex were suggested as other potential determinants of interindividual variation in plasma concentrations of enterolignans(Reference Halldin, Eriksen and Brunius46).
Metabolomic approach for a metabotyping based on absorption, distribution, metabolism and excretion capacity
Subjects presenting similar metabolic capacity towards plant food bioactives can be grouped into metabotypes. The stratification of individuals into different metabotypes is emphasised by the COST-POSITIVe network as a potential strategy to identify population subgroups that could gain particular benefit from the consumption of specific plant food bioactives.
Targeted metabolomics, largely used for quantitative bioavailability studies, appears as a valid approach for stratification of individuals depending on their ability to produce specific metabolites defining a specific aspect of the metabotypes(Reference Tomas-Barberan, Garcia-Villalba and Gonzalez-Sarrias40). Besides, an untargeted metabolomic approach provides the advantage to obtain a comprehensive phenotype of the internal exposure, which embraces the diversity of the metabolites of food phytochemicals(Reference Koistinen, da Silva and Abranko47–Reference Ulaszewska, Weinert and Trimigno49). This wide profiling of metabolites can be used both a posteriori to stratify or classify subjects according to their internal exposure to phytochemical metabolites, and also to prospectively establish correlations between metabolites signatures and health-related outcomes(Reference Gonzalez-Sarrias, Garcia-Villalba and Romo-Vaquero42,Reference Kreijkamp-Kaspers, Kok and Bots50 ). However, moving in that direction requires improvements in the methodology and tools. The COST-POSITIVe network has assessed the current limitations of the approach and discussed the possibilities to optimise and harmonise untargeted methods of analysis based on MS. More specifically, the network has organised a multiplatform test comparing the analytical coverage of existing methods applied across Europe, which has provided insights for optimisation and harmonisation of methods ensuring sufficient sensitivity and wide coverage of plant food bioactives and their metabolites in biological samples(Reference Koistinen, da Silva and Abranko47). The pursuit of these collaborative developments would facilitate the comparison of exposure profiles in independent studies, including large population studies with associated data on age, sex and other factors of interest.
COST-POSITIVe has also contributed to the improvement of online databases and open-access tools of special interest for researchers working in the field of plant food bioactives and their health benefits. For example, as previously reported, the PhytoHub database (http://phytohub.eu/), conceived to facilitate the identification of food phytochemicals and their metabolites in metabolomic profiles, has been updated by COST-POSITIVe experts(Reference Landberg, Manach and Kerckhof29). Partners have also enriched the collaborative platform FoodComEx (http://foodcomex.org/) for the sharing of authentic standards particularly useful for the validation of compound identification in untargeted metabolomics. From the chromatographic data provided by COST-POSITIVe partners and collaborators, the usefulness of the online tool PredRet, designed to predict the retention times of compounds(Reference Stanstrup, Neumann and Vrhovsek51), has been demonstrated to help in the identification of plant food derived metabolites.
Interindividual variation in biomarkers of cardiometabolic health in response to plant food bioactives
Only a limited number of trials have suggested that beyond differences in bioavailability, particular metabolic or related disease traits of individuals can influence their cardiometabolic response to food phytochemicals(Reference Milenkovic, Morand and Cassidy27). To address this issue, the COST-POSITIVe network systematically analysed data from published studies to assess interindividual variation in selected clinical biomarkers associated with cardiometabolic risk, including blood lipids, endothelial function, blood pressure and glucose metabolism, in response to the consumption of various categories of plant food bioactives. Wherever possible, a range of intrinsic factors has been assessed (e.g. age, sex, BMI, health status, ethnicity, genetic background and gut microbiome) as potential determinants of the between-subject variations in the cardiometabolic response to the consumption of flavonoids (flavonols, flavanols and anthocyanins), ellagitannins, phenolic acids and plant sterols.
As recently described(Reference Gibney, Milenkovic and Combet28), the strategy of the COST-POSITIVe network was to conduct for the first time extensive literature and data analysis from the published RCT, leading to the execution of several meta-analyses published or under preparation. These meta-analyses were subsequently used, where possible, to perform subgroup analysis and/or meta-regression based on selected factors in order to determine their influence on variability. The ultimate goal was to identify the determinants, beyond bioavailability, genetics and gut microbiota, responsible for most of the variability in the cardiometabolic outcomes reported. Most of the published RCT analysed have used phytochemical-rich or enriched foods rather than pure compounds. This has led to performing meta-analyses focusing on flavanol-containing tea, cocoa and apple, on anthocyanin-rich and ellagitannin-rich foods, and plant sterols enriched products. The resulting systematic reviews and meta-analyses consistently show that all these phytochemical-rich foods can improve some cardiometabolic endpoints as detailed previously. Briefly, the major conclusions of the meta-analysis completed and published so far were that: (i) the consumption of flavonol-rich food can improve blood cholesterol profiles(Reference Gonzales-Sarrias, Pinto and Mena21), (ii) that ellagitannins- and anthocyanin-rich foods may also reduce total cholesterol and blood pressure(Reference Garcia-Conesa, Chambers and Combet20), and (iii) that flavonol supplementation slightly improves blood lipids, fasting glucose and reduces blood pressure(Reference Menezes, Rodriguez-Mateos and Kaltsatou22). From the subgroup analysis, it appears that the efficacy by which these flavonoid and non-flavonoid compounds (ellagitannins) improve cardiometabolic risk biomarkers is highest in those with a high BMI, a result that needs to be confirmed in prospective intervention studies. It was not possible to assess the role of other factors such as age, sex, ethnicity and lifestyle (smoking and dietary habits) in the variability of the biological responsiveness to these compounds, based on available data. Another systematic review has focused on the main factors of interindividual variability in biomarkers of cardiometabolic health in response to the intake of hydroxycinnamic-rich foods or extracts. This analysis demonstrated that regardless of the source of hydroxycinnamic acids, the greater effectiveness was observed in subjects at higher risk of cardiometabolic disease(Reference Martini, Chiavaroli and Gonzalez-Sarrias52).
It is well known that supplementation with phytosterols causes substantial variability in the individual LDL-cholesterol response. This is mainly explained by several polymorphisms affecting genes involved in the cholesterol-trafficking pathways(Reference Jones25). Beyond these genetic factors, the systematic analysis of RCT focused on the effects of the consumption of foods fortified with plant sterols on cardiometabolic outcomes conducted by the COST-POSITIVe network indicated dose-dependent and consistent positive changes in the Apo profile. This effect was not dependent on sex, but the improvement appeared higher in younger (<40 years) study populations(Reference Gibney, Milenkovic and Combet28).
The systematic work conducted by the COST-POSITIVe network clearly highlighted that the available dataset from RCT is most often not adequate to properly assess the factors that could explain the interindividual variability in responsiveness. We found a large discrepancy in the reporting quality of the studies analysed, and no consensus in the description of the population characteristics or the applied statistical methodologies. This hampered both the possibility and the quality of subgroup analysis that are required to identify major determinants of interindividual variability. The lack of access to individual datasets of published studies also constitutes a major limitation as it prevents opportunities for data fusion, which would constitute the best approach for an accurate assessment of the factors of variability.
Together with its crucial role in the absorption and metabolism of several plant food bioactives, the COST-POSITIVe network has also highlighted the importance of considering more specifically, the role of the gut microbiota in the variability of the biological response. Depending on its composition and functionality, the gut microbiome can produce some bioactive metabolites (e.g. equol and urolithins) that seem able to affect some cardiometabolic outcomes leading to different responses depending on the metabotypes(Reference Gonzalez-Sarrias, Garcia-Villalba and Romo-Vaquero42,Reference Hazim, Curtis and Schar53 ). However, the beneficial effects may also be produced by the specific gut microbial community associated with the production of these microbial metabolites(Reference Ozdal, Sela and Xiao54). In that case, metabotypes can reflect the gut microbiota composition and metabolic status and could be considered as biomarkers of the potential polyphenol health effects mediated through gut microbiota(Reference Tomas-Barberan, Selma and Espin55). In any case, these uncertainties strengthen the need to develop research aiming to clarify the reciprocal interrelations between the gut microbiota and food bioactives.
Assessment of the variability of the genomic response to plant food bioactives intake
Exposure to food phytochemicals has been shown to promote in vitro and in vivo changes at the level of gene expression and signalling pathways that ultimately modulate key biological processes involved in the regulation of cardiometabolic health(Reference Krga, Milenkovic and Morand13,Reference Upadhyay and Dixit14 ). Enhancing our knowledge of the cellular and molecular targets involved in the mediation of the cardiometabolic effects of plant food bioactives is essential for a better understanding of the mechanisms of action of these compounds and also of the variability in their biological responsiveness. To this end, the COST-POSITIVe network conducted a systematic analysis of the reported genomic effects of food phytochemicals, focusing efforts on compounds for which the cardiometabolic effects and related mechanisms were particularly well documented. The strategy was: (i) to use bioinformatic tools to establish a list of key genes involved in the regulation of cardiometabolic health for which expression had been reported to be modulated by the compounds of interest in in vivo and physiologically relevant in vitro studies, (ii) to analyse these genes for protein–protein interactions to identify genes in the nodes of the interaction network, and (iii) to search in the SNP databases to identify and select polymorphisms that may have an impact on the expression and/or regulation of these genes(Reference Gibney, Milenkovic and Combet28). It was assumed that if these polymorphisms existed, they could potentially influence the molecular response to plant food bioactives and consequently contribute to the variability of the biological responsiveness to their intake. As such, it would become relevant to identify and then investigate these polymorphisms in future nutrigenetic studies. To date, COST-POSITIVe partners have applied this strategy to flavanols, and the results are being prepared for publication.
COST-POSITIVe has also compiled and critically analysed human subjects’ intervention studies that have evaluated how changes in gene expression can be attributed to different categories of plant food bioactives (polyphenols, glucosinolates and plant sterols)(Reference Pokimica and Garcia-Conesa56). This analysis revealed that studies often suffer from a range of limitations, notably with regard to inappropriate study designs, experimental settings and data analysis. Some studies provide poor evidence to support gene–target interactions. To overcome these limitations and increase the quality of future studies, the need to apply normalised procedures and follow some methodological guidelines has been highlighted. This would improve the reliability and biological relevance of results for a better understanding of the molecular responses to plant food bioactives and also of the interindividual variability in the response to their intake.
Gaps in knowledge and future research needs
The COST-POSITIVe group is convinced that a better knowledge of the efficacy of plant bioactives via a full understanding of the interindividual variability in the bioavailability and biological response to the consumption of these compounds can help to optimise dietary recommendations and food products development especially designated for specific population subgroups. However, the work carried out within the network highlighted the difficulties in assessing the between-subject variation in the bioavailability and biological responsiveness to plant food bioactives intake from existing studies. Information on the different factors for relevance to between-subject variation has typically not been reported.
Up to now, the nutrition and food research community has not implemented the appropriate study designs for intervention trials with bioactives that would allow the analysis of the interindividual variability, as well as of the factors affecting it. Indeed, most published studies have presented their results using classic statistical estimators of the sample population distribution, i.e. mean or median values with or without data variance thereby masking the real range of effect differences (size and direction) between the individuals(Reference Morand and Tomas-Barberan57). In addition, available studies have most often a very small sample size and thus, it was not possible to stratify individuals based on their responsiveness with sufficient statistical power to allow the clear identification of the factors influencing the variability and/or of the subgroups responding more homogeneously. Taking into consideration the above limitations and the need to improve the quality of the reporting of human subjects’ responses to dietary intervention, the COST-POSITIVe group has developed and validated a quality index score, the so-called POSITIVe quality index. This score incorporates various criteria regarding the statistics, the reporting and presentation of data and the availability of individual data(Reference Nikolic, Konic Ristic and Gonzalez-Sarrias58). Consideration of this POSITIVe index will guide researchers, peer-reviewers and journal editors to assess the quality of the reporting in published or submitted papers and will help to improve the design and conduct of further trials focusing on interindividual variability in response to interventions with bioactive compounds.
Attempts to combine and analyse individual datasets across some studies made available from COST-POSITIVe partners have also failed to provide sufficient information to definitively identify which factors are really responsible for interindividual variability. This was mainly due to the small number of available studies and the difficulty to merge data from studies that differ in study designs and outcomes. Despite the current lack of sufficient high-quality data for data fusion analyses, this strategy constitutes a relevant approach that would bring together large datasets and would facilitate subgroup analyses by providing sufficient statistical power. This would help to identify the key factors driving responsiveness in individuals or, at least, in groups of subjects sharing similar characteristics. The COST-POSITIVe network has also experienced that this data fusion approach is inherently complex because of ethical limitations relating to getting access to data(Reference Gibney, Milenkovic and Combet28). Hopefully, this issue will be partly alleviated with the growing number of academic research calls asking for more availability of the data generated within the funded projects. Databases designed to collect all these individuals' data for each individual and to allow for data fusion, stratification and analyses of large sets of results would provide an essential contribution to identify how and which factors influence the ADME and biological responsiveness to dietary plant bioactives.
Between-subjects differences in ADME are believed to underpin much of the interindividual variability in the response to plant bioactive compounds. It may reflect differences in human subjects’ genetics regarding digestive enzymes, intestinal transporters, phase I and II metabolism or tissue carriers, but also differences in gut microbiota composition and functionality affecting the catabolism of compounds not absorbed in the small intestine. However, the key molecular determinants of ADME variability for the different categories of food phytochemicals remained to be established. An additional and very important need is to establish the potential association between the exposure to the different metabolites available to the organism (i.e. biological fluids and tissues) and the changes in the specific cardiometabolic biomarkers observed after the intake of a source of plant bioactives. Correlations between bioavailable compounds and changes in cardiometabolic biomarkers within the same individuals would help to support a cause–effect relationship, to envisage potential mechanisms of action in vivo, and to better stratify the individuals based both on internal exposure and response. Such correlations will also help to determine the contribution of ADME in the interindividual variability of the biological responses. However, studies providing data on both the bioavailability and biological responsiveness to plant bioactives are still very few and we herein reinforce the need for conducting more studies in the future(Reference Morand and Tomas-Barberan57).
To capture interindividual variation in response to the consumption of the main families of plant food bioactives, COST-POSITIVe highlights the need for a new generation of specifically designed intervention studies with an in-depth phenotyping of the individuals, assisted with omics technologies(Reference Bayram, Gonzalez-Sarrias and Istas59), and appropriate statistical approaches to analyse interindividual variability and allowing data aggregation. These studies will rely on: (i) development of food metabolome databases, (ii) use and integration of omics data, including genotyping of relevant genes, metabolomic profiling with standardised and reliable methods to characterise the individual metabolic capacity and metagenomic profiling, (iii) the collection of biological samples to examine both bioavailability/internal exposure and biological responsiveness using well-established biomarkers of health and (iv) on the collection of data related to lifestyle. To allow the fusion and integration of data between studies, it would also be of special relevance to develop new and standardised protocols to assess jointly the individual's capacity to: (1) absorb and/or metabolise the plant food bioactives (e.g. challenge test approach using a standardised supplement containing various compounds) and (2) respond to the intake of those compounds (e.g. selecting conditions most suitable to reveal clinically relevant changes in biological outcome assessed). This will provide the right ‘canvas’ and generate appropriate data to build up a more robust causal relationship, as well as to determine the contribution of different factors (e.g. sex, age, BMI, genetic polymorphisms, gut microbiota, pathophysiological status, smoking, physical activity and dietary habits) that may influence the interindividual variability for the different families of plant food bioactives.
Another interesting option raised by the COST-POSITIVe network would be to investigate the extent of the interindividual variability in cohort studies that allow multiple longitudinal samplings and metabolic profiling of blood, urine and gut microbiome to ascertain the drivers of the individual responsiveness for cardiometabolic risk factors(Reference de Roos, Aura and Bronze60).
Conclusion
The results from the COST-POSITIVe highlight that an optimisation of the health benefits associated with plant food consumption requires the identification of individuals or population subgroups that would more likely benefit from increased intake of plant food bioactives. This will be only achieved by increasing our knowledge of the main determinants of the interindividual variability of ADME of plant bioactive compounds but also of the biological responses to phytochemical-rich foods (Fig. 2). Gaining knowledge in the field implies to carry out a new generation of human subjects’ intervention studies using the conceptual and methodological approaches suitable to analyse variability in response to plant bioactives intake.

Fig. 2. (Colour online) POSITIVe-COST Action (2015–2018): interindividual variation in response to consumption of plant food bioactives and determinants involved. The large magnitude of the interindividual variation observed, in both the absorption/metabolism and in the changes in biomarkers of cardiometabolic risk in response to consumption of many families of plant bioactives, may reflect between-subjects differences in a diversity of intrinsic and extrinsic factors that remain to be identified. The identification of these main determinants of interindividual variation for the major families of bioactive compounds will allow a better understanding of their health effects, as well as the development of tailored dietary advice and functional foods for groups of people sharing the same metabolic capacity (metabotypes) and/or biological responsiveness. Ultimately, this knowledge will allow moving from the ‘one-size-fits all’ approach towards a science-based personalised nutrition approach focusing on plant foods rich in specific bioactive compounds, with a higher impact on the prevention of cardiometabolic diseases.
A clear understanding of why some plant bioactive compounds may be highly effective in some individuals but less or not in others will help to refine the recommendations of plant foods rich in specific bioactives. This information will become very useful to public health authorities to further develop and implement health policies and refined dietary advice to improve the cardiometabolic health of populations. In addition, this knowledge is also crucial to develop a scientific basis to underpin the development of new functional foods or optimised traditional foods with demonstrated health benefits for targeted groups of consumers. Moving towards a science-based personalised nutrition approach focusing on plant foods rich in specific phytochemicals could be of special interest to help in tackling the burden of metabolic and CVD.
Acknowledgements
This article is based upon work from COST Action FA1403 POSITIVe (interindividual variation in response to consumption of plant food bioactives and determinants involved) supported by COST https://www.cost.eu). The authors also acknowledge all the partners of the COST Action POSITIVe for their involvement in the network and their key contribution to the advancement in the field covered by the action.
Financial Support
This work received financial support from Cost Action FA1403 – European Cooperation in Science and Technology.
Conflict of Interest
None.
Authorship
Ch. M. and F. T. B. conceived and drafted the manuscript. All the authors contributed with critical intellectual input, read and revised the final draft.