Three-dimensional (3D) food printing technology can fabricate food objects layer by layer, from the bottom to the top. The technology can design geometries of food objects guided by computer-aided design models or scanned 3D models(Reference Sun, Zhou and Huang1). 3D food printing is recognised as a new frontier in the food industry to enable rapid prototyping, customised food design and personalised nutrition(Reference Sun, Zhou and Huang1). Furthermore, as the world is becoming food and nutrition insecure due to unstable world food supply chains and climate variability, 3D food printers as home appliances could serve as more inclusive and affordable tools to deliver personalised nutrition through localised food supplies and food waste upcycling(2).
There has been an explosion of 3D food printing publications since 2008, as revealed by bibliometric analyses(Reference Fasogbon and Adebo3–Reference Baiano5). While the applications of such technology are far-reaching, its potential to benefit vulnerable communities with chewing and swallowing difficulties by reshaping texture-modified foods (TMF) is exceptionally appealing(Reference Baiano5). Nevertheless, the concepts of using 3D food printing for personalised nutrition and reshaping TMF are often impractical, with multiple inherent technological constraints, as discussed in this review. Furthermore, with 3D food printing in its infancy, there are unknown impacts on the food system and on human health(Reference Sun, Zhou and Yan6,Reference Derossi, Bhandari and van Bommel7) . This review focuses on extrusion-based 3D food printing, the most common 3D food printing technology(Reference Baiano5), and depicts its current readiness in real-world applications, particularly in human nutrition and health. We highlight the barriers to implementation and means to overcome these to enable the deployment of 3D food printing to improve food and nutrition security and sustainability. Finally, we discuss consumer acceptance, ethical and regulatory requirements and the cost-effectiveness of 3D food printing, which can ultimately determine its adoption and sustainability.
State-of-the-art of extrusion-based three-dimensional food printing
There are three types of 3D food printing technology: extrusion-based printing (including melt extrusion deposition), powder bed-based printing (including selective laser sintering printing, selective heat sintering and binder jetting) and inkjet printing (Table 1)(Reference Sun, Zhou and Huang1,Reference Baiano5) . Among them, extrusion-based 3D food printers are the most common due to their relatively simple operation, easy material handling, and compatibility with a wide range of food materials(Reference Saadi, Maguire and Pottackal8). Commercial desk-top 3D food printers are evolving rapidly and becoming faster, more affordable, precise, and user-friendly(Reference Sun, Zhou and Huang1). Moreover, many international food companies (e.g. Redefine Meat, Barry Callebaut, PHILIPS, Barilla, Nestle, Hershey, Mondelez and PepsiCo) have invested in 3D food printing(Reference Rubio, Hurtado, Godoi, Bhandari, Prakash and Zhang9,Reference Linden10) .
Table 1. Three-dimensional food printing technologies and commercial three-dimensional food printers

Food materials and processing for extrusion-based three-dimensional food printing
Many reviews have discussed food materials that can be used for extrusion-based 3D food printing(Reference Sun, Zhou and Huang1,Reference Voon, An and Wong11) . In theory, extrusion-based 3D food printing is versatile enough to print a wide range of food materials, such as chocolate, fats, dough, pureed or mashed fruits and vegetables, edible gels (hydrocolloids, gums, starch and protein), hummus, creamy cheese, icing, spread, surimi and meat slurry(Reference Liu, Zhang and Bhandari12,Reference Kim, Bae and Park13) . Some novel food materials and ingredients, such as proteins from insects, algae and fungi, are also printable(Reference Bedoya, Montoya and Tabilo-Munizaga14,Reference Zhao, Zhang and Chitrakar15) . Typically, these materials should flow through a nozzle and are self-supporting after being deposited on a surface. However, not all materials and formulations are directly printable. The substantial variations in the composition and physico-chemical properties of food materials within and across batches can result in low-printing repeatability and reproducibility(Reference Severini, Derossi and Ricci16). Existing 3D food printing research generally adopts experimental food materials, such as hydrogel (food gums) and starch-based systems (e.g. mashed potato or rice), which typically are not ready for human consumption and are distinct from real food systems during 3D food printing(Reference Pereira, Barroso and Gil17).
The material's rheological properties are fundamental to the successful processing and printing of food. In addition, the physico-chemical properties (e.g. water holding capacity, syneresis), rheological properties and compositional profiles of pre-cooked food inks will change during storage(Reference Diamante and Umemoto18). For example, pre-prepared dough is generally printable only within 1–2 h(Reference Lipton, Cutler and Nigl19). An alternative solution is to develop food material-specific printers, such as chocolate printers (Table 1). However, such an approach overlooks the versatility of the technology. Instead, users should be provided with multifunctional ‘plug and print’ printers with capacity to print a wide variety of common food materials.
Three-dimensional geometric design and software
The 3D geometric design is a critical but often overlooked component in 3D food printing. In many instances, building printable 3D models itself is extremely time-consuming, requiring multiple software, including 3D modelling software (e.g. SketchUp and blender) and slicing software (e.g. Ultimaker Cura and Slic3r), all of which require users to have extensive experience and skills in graphic design and editing mesh or stereolithography data. Many online 3D model repositories provide pre-built 3D models (e.g. Thingiverse, Cults, Thangs and Printables)(Reference Guo, Zhang and Bhandari20). However, most online 3D models require further modifications (e.g. removing small features in the models, closing mesh holes and gaps, and resolving non-manifold geometry), which is time-consuming and laborious also.
There is no ‘one-size-fits-all’ solution when matching printing parameters and 3D models. Instead, other 3D food printing components (i.e. food material, printing parameters and post-printing process) should be taken into consideration when building 3D models to maximise printing performance. For example, extrusion-based 3D food printing requires soft extrudable food material; therefore, the 3D shapes after printing should be self-supporting. Furthermore, nozzle size, slicing method (layer height) and the capacity of printers can dominate the fidelity of the printed construct, which should be reflected in the 3D model designing process(Reference Nijdam, Agarwal and Schon21,Reference Hao, Mellor and Seaman22) . In parallel, other printing parameters such as nozzle retraction, toolpath (i.e. motion trajectory of the nozzle), infill density and pattern (e.g. grid, spiral, concentric or zig-zag) and printing temperature should be tuned for each specific material and 3D shape(Reference Chen, Zhang and Teng23). Collectively, 3D geometric design for 3D food printing involves multiple software and deep knowledge of numerous factors in the printing process. Therefore, requiring general consumers to build their own 3D models is currently impractical. In this context, existing smartphone photogrammetry and 3D scanning apps (e.g. RealityCapture) could be calibrated to generate 3D mesh models ready for 3D food printing. Generative artificial intelligence algorithms that can create 3D shapes using easy 2D images (e.g. NVIDIA 3D MoMa) or even descriptive texts (e.g. dreamfields3D, and ChatGPT-powered 3D modelling editors such as blender and Unity) could be further tuned for 3D food printing.
Post-printing processing
Post-processing can increase stability (stiffness), and improve food safety and shelf life, aesthetics and palatability. However, post-printing processing of 3D-printed food products is challenging due to the low mechanical strength of the food materials(Reference Phuhongsung, Zhang and Devahastin24). Conventional heat processing such as baking, toasting, frying, microwaving and steaming can decrease the fidelity of prints and lead to shrinkage, cooking loss, colour changes and texture shifting(Reference Dick, Bhandari and Prakash25). Extrusion-printed 3D foods, such as soft and pureed foods, are typically unsuitable for reheating and long-term storage because their shapes can collapse, and cause water leakages. 3D-printed foods are generally expected to be consumed immediately after printing. As a result, food additives (e.g. gums, methylcellulose, protein isolate and gelatin, starch and modified starch, calcium chloride and calcium caseinate) are often used to improve the stability of the prints during post-processing(Reference Phuhongsung, Zhang and Devahastin24,Reference Hussain, Malakar and Arora26) . Some of these components may adversely impact the nutritional quality of 3D-printed foods. By contrast, freeze-drying is a viable alternative for maintaining the structure of these printed products.
Current key barriers to adopting three-dimensional food printing in real-world applications
Low-printing speed is the key bottleneck in real-world applications of 3D food printing(Reference Sun, Zhou and Huang1,Reference Verma, Kamble and Ganapathy27) . This is particularly evident in some settings (e.g. restaurants and residential aged care facilities) where many meals need to be prepared within a short timeframe. Some 3D food printers are optimised for specific food materials and use higher-quality components to improve print head motion, increasing the printing speed. For example, the Netherlands Organisation for Applied Scientific Research is developing multi-nozzle, high-force and high-speed extrusion printers. Another way to accelerate 3D food printing is to reduce printing size (portion size). However, food inks should be enriched to achieve the required nutrient provision. In addition, commercial 3D food printers require users to fill the food capsules (cartridges) manually, which is far from efficient. Therefore, users should be provided with standardised, ready-to-print, pre-filled food capsules (ink cartridges) that enable ‘plug and print’.
Poor repeatability and reliability of printing are other challenges(Reference Sun, Zhou and Huang1). 3D food printers require intensive monitoring during printing. Improper control of the printing parameters and 3D design may lead to various defects, including nozzle blockage, inconsistent extrusion, layer shifting, material spreading, insufficient retractions and ‘elephant foot’ (i.e. the first few layers are larger than the others due to food weight), or the printed object collapsing. Having fully autonomous printers that can resolve common defects will reduce human supervision, making 3D printing more reliable and convenient. To this end, artificial intelligence-based in-situ detection and real-time printing correction can be critical to achieve high 3D printing performance(Reference Goh, Sing and Yeong28–Reference Ma, Potappel and Chauhan30). In a recent study, Ma et al.(Reference Ma, Potappel and Chauhan30) used computer vision to track the extrusion process and optimise the extrusion rate and nozzle motion.
Lastly, 3D food printing can be a ‘double-edged sword’. As shown in Table 1, many commercially available 3D food printers are designed for chocolate and sugar printing. Chocolate and sugar are hot-melt materials that melt into a liquid form upon heating and solidify quickly into a self-supporting object after cooling(Reference Periard, Schaal and Schaal31). 3D-printed chocolate and sugar products can have ‘eye-catching’ shapes, which have been used to introduce and advertise 3D food printing in many news coverages and studies(Reference Lupton32). These eye-catching shapes could negatively affect consumers' ‘first impressions’ of 3D-printed foods and encourage poor nutritional choices, particularly in the younger generation(Reference Kearney, Fitzgerald and Burnside33). Moreover, it is worth noting that 3D-printed chocolate and sugar products and many other printed cereal-based foods could be considered as ultra-processed food products(Reference Srour, Kordahi and Bonazzi34). Numerous epidemiological and clinical studies have suggested an association between ultra-processed food consumption and various cancers and chronic diseases(Reference Srour, Kordahi and Bonazzi34). As discussed further later, the previously described challenges highlight the need for explicit guidelines to direct 3D food printing-related academic and industrial practices to minimise the negative effects of the technology(Reference Lupton32).
Adoption of three-dimensional food printing to promote healthy and sustainable eating
The recent research boom in 3D food printing has been driven by the broad array of applications, in personalised nutrition particularly(Reference Baiano5). However, the personalised nutrition field is in its infancy, with many proposed applications still based on insufficient and inconclusive scientific evidence(Reference Ordovas, Ferguson and Tai35). Instead, in this review we aim to identify feasible applications for 3D food printing that would be achievable and implementable in the near future to promote healthy and sustainable eating.
Redesigning texture-modified foods for vulnerable communities
Using 3D food printing technology to reshape TMF is an important emerging application. Speech and language therapists prescribe TMF – soft, moist, minced, pureed or liquidised foods – for people with chewing and swallowing difficulties (dysphagia). The prevalence of swallowing difficulties is estimated to be about 8 % globally(Reference Cichero, Steele and Duivestein36). The International Dysphagia Diet Standardisation Initiative framework defined a hierarchy of seven texture levels for TMF and fluids(37), which is similar to the ‘Smile Care Food’ system in Japan (Table 2)(Reference Maksimenko, Lyude and Nishiumi38). In contrast, the USA(Reference Nishinari, Turcanu and Nakauma39), Japan and Canada(Reference Cichero, Steele and Duivestein36) use instrumental texture and rheological measurements (i.e. yield stress, hardness and viscosity) as indicators to classify TMF. However, despite their increasing use, the provision of TMF in many care settings (including aged care, hospital care and home care) has remained underdeveloped(Reference Miles, Liang and Sekula40). For example, TMF are commonly served as ‘ice cream balls’ because of the portion scoops. In addition, those on TMF diets face persistent and severely restrictive food varieties, representing a silent food insecurity issue for these vulnerable people(Reference Burger, Kiesswetter and Alber41–Reference Abbey, Wright and Capra43). The transition to TMF from standard diets leads to reduced appetite(Reference Shimizu, Fujishima and Maeda44); lower intakes of vitamins A and E(Reference Mann, Heuberger and Wong45), protein and fluid(Reference Bannerman and McDermott46) and higher weight loss(Reference Painter, Le Couteur and Waite42). Unsurprisingly, the habitual consumption of TMF is often linked with malnutrition (undernutrition)(Reference Vucea, Keller and Morrison47,Reference Wu, Miles and Braakhuis48) .
Table 2. International Dysphagia Diet Standardisation Initiative (IDDSI), the National Dysphagia Diet (NDD) USA and ‘Smile Care Food’ Japan terminologies for texture-modified foods and drinks
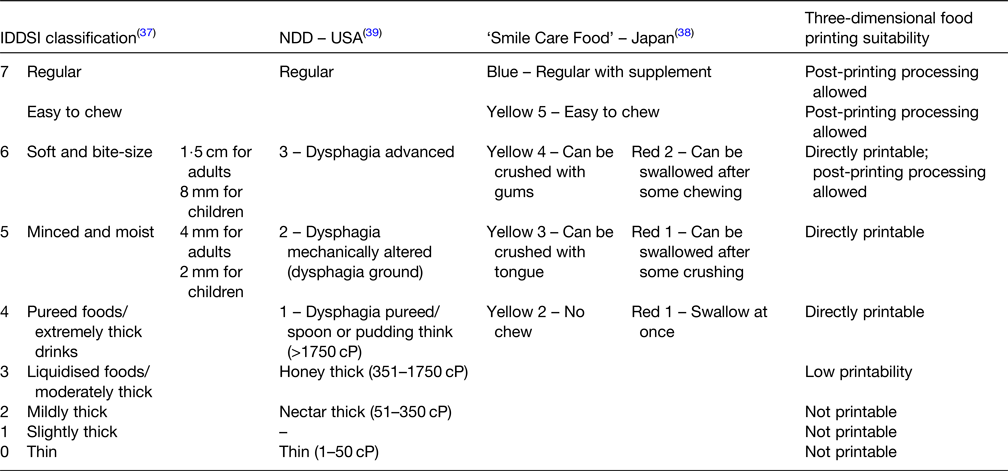
cP, centipoise; measured at shear rate of 50/s and 25°C.
The physical properties of many TMF are the same as the food materials required for extrusion-based 3D food printing. Therefore, TMF could be suited to 3D food printing(Reference Baiano5,Reference Sun, Zhou and Yan6,Reference Zhang, Pandya and McClements49) . In turn, 3D food printing could address the multiple interacting drawbacks about TMF(Reference Steele, Alsanei and Ayanikalath50,Reference Lorenz, Iskandar and Baeghbali51) . Firstly, 3D food printing may improve food intake by aged care residents by developing aesthetically appealing food options (Fig. 1)(Reference Baiano5). However, studies indicated that shapes should be carefully selected based on consumer demographics and application scenarios. In a recent example, allied health professionals suggested that 3D-printed food products for aged care residents should be in the original food shape to help consumers ‘recognise the food item’ and match their fellow dinners (‘look like everybody else's food’)(Reference Smith, Bryant and Hemsley52–Reference Zhong, Adu and Devine54). Using non-food-like shapes such as geckos or flowers could be ‘childish’ and embarrass the person on TMF(Reference Smith, Bryant and Hemsley52,Reference Keller and Duizer53) . Therefore, population- and context-specific 3D geometries should be considered. Secondly, detection thresholds among older people for salt, sour, sweet, umami and bitter tastes may increase, therefore lowering their food enjoyment(Reference Doets and Kremer55). 3D food printing can enhance nutrition by personalising or medically tailoring the food inks to meet these demands, thus enhancing palatability. The same strategy can benefit various other vulnerable groups (e.g. people with motor neurone disease or multiple sclerosis; children with cerebral palsy, acute hospital care and rehabilitation patients such as stroke survivors).
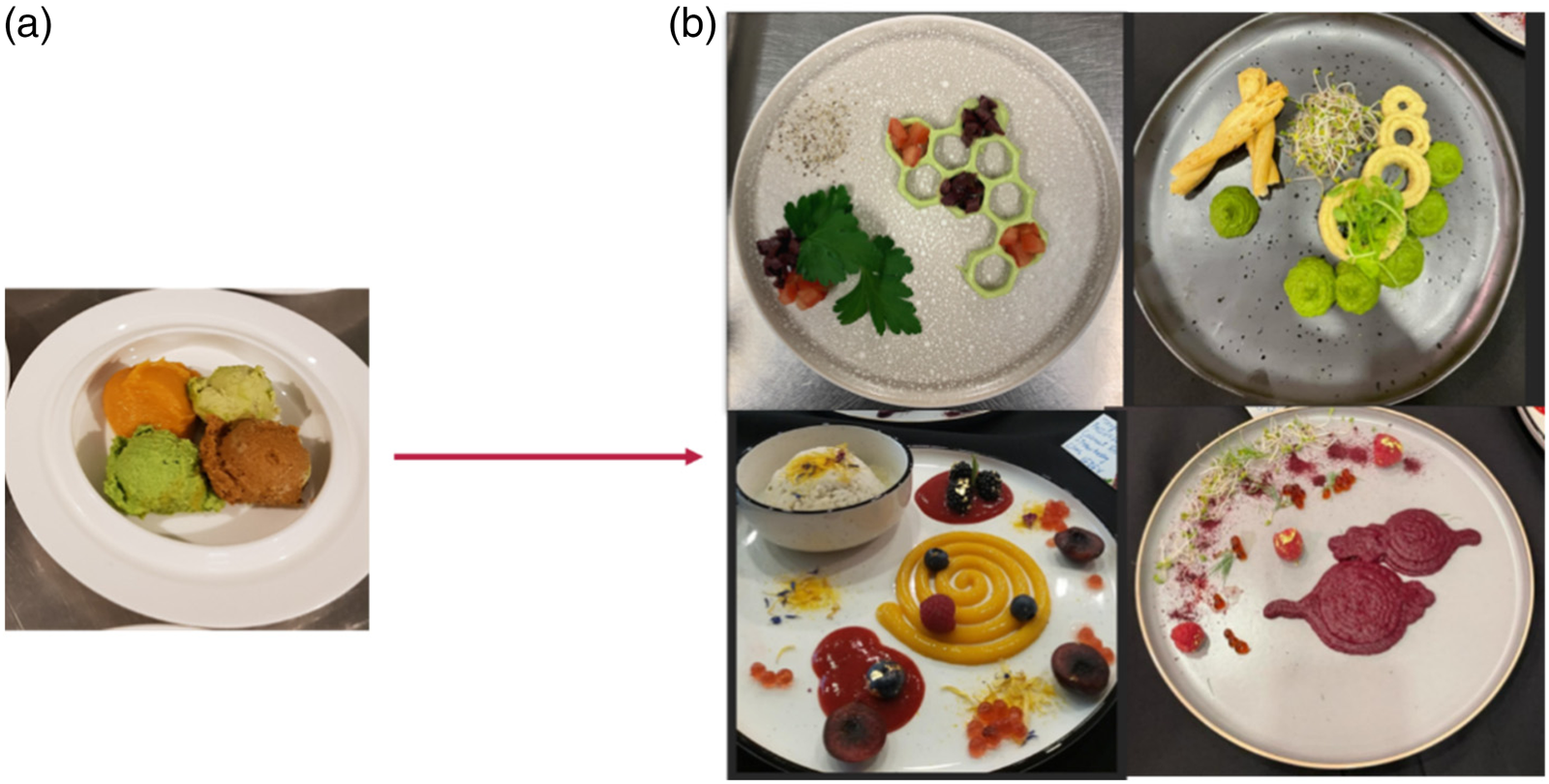
Fig. 1. Conventional texture-modified food presented as unattractive ‘dollops’ (a) and three-dimensional-printed meals (b).
Hospitals and aged care institutions commonly use cook–chill food service systems, which involve a series of food processing, including packaging, refrigerating, delivery, storage, transportation and reheating. The impacts of different food processing on the printability of food materials and the stability of printed products should be carefully examined(Reference Zhao, Zhang and Chitrakar15,Reference Dick, Bhandari and Prakash25) . Lastly, aged care facilities have extremely constrained food and nutrition budgets. The extra costs in equipment, staff and training for 3D food printing could further increase health disparities among communities on TMF(Reference Timmermans and Kaufman56). Overall, while providing 3D-printed foods with high nutritional value to vulnerable individuals could enhance health outcomes, there is little original research to support the nutritional benefits of 3D food printing(Reference Hemsley, Palmer and Kouzani57). The European Union funded the PERFORMANCE (Development of Personalised Food using Rapid Manufacturing for the Nutrition of Elderly Consumers) project in 2012. It was the first 3D food printing project to develop 3D-printed foods specifically for aged care residents, however, no published results related to 3D food printing was found(58,Reference Rusu, Randriambelonoro and Perrin59) .
Three-dimensional food printing to enhance nutrition literacy
3D printing technology is built on the multi-discipline of science, technology, engineering and mathematics, therefore, can be a novel tool in teaching and education(Reference Ford and Minshall60). Notably, 3D printing has been used for special education, for example, groups with cognitive, motor and visual impairments(Reference Buehler, Comrie and Hofmann61). Similarly, in addition to health-related applications, 3D food printing technology can offer a unique opportunity for education purposes, as a captivating education tool for food and nutrition literacy, for example, with its visualisation, excitement and creativity to promote healthy eating(Reference Gosine, Kean and Parsons62). However, little has been published on applying 3D food printing in food and nutrition education. Gosine et al.(Reference Gosine, Kean and Parsons62) performed focus groups with dietitians, teachers and nutrition students to explore their insights on applying 3D food printing in nutrition education. The study stated that ‘the participants did not feel that a 3D food printer would enhance their teaching and instead felt it could confuse or frighten people’(Reference Gosine, Kean and Parsons62). Nevertheless, the nutrition students expected that 3D food printing could trigger ‘higher engagement in the food science courses’(Reference Zhong, Adu and Devine54).
Food upcycling
Due to its unique flexibility in reshaping food materials, 3D food printing has been explored for its potential to use multiple by-product wastes and novel food materials (e.g. insects)(Reference Bedoya, Montoya and Tabilo-Munizaga14,Reference Zhao, Zhang and Chitrakar15) . For example, by-product waste from potato processing(Reference Muthurajan, Veeramani and Rahul63,Reference Feng, Zhang and Bhandari64) , grape pomace waste from wine and juice production, broken wheat from the milling industry(Reference Jagadiswaran, Alagarasan and Palanivelu65), low-value minced meat offcuts(Reference Rubinsky66) and seafood (e.g. salmon, cod and crab)(Reference Carvajal-Mena, Tabilo-Munizaga and Pérez-Won67–Reference Kim, Kim and Park69) have been value-added into high-value food products using laboratory 3D food printing. 3D food printing provides the avenue to transform aesthetically imperfect or unsold fruits and vegetables that otherwise often end in landfill into high-value human foods(Reference Baiano5). To this end, 3D food printing can be a powerful tool to fight against food waste, while aesthetically imperfect but perfectly edible fresh produce can result from climate change due to climate variability and food supply chain disruption(70).
Sensory manipulations using three-dimensional food printing to promote healthy eating
Beyond formation of shapes, 3D food printing can also mask or enhance food colour(Reference Kewuyemi, Kesa and Meijboom71), aroma, taste(Reference Fahmy, Amann and Dunkel72) and mouthfeel(Reference Tabriz, Fullbrook and Vilain73). For example, increasing the surface-to-volume ratio of a 3D-printed dough object by adjusting infilling density and pattern can accelerate the baking process and alter the texture properties (such as hardness) and mouthfeel (e.g. crispy, sticky, crunchy and smooth) of final products(Reference Zhang, Lou and Schutyser74). Similarly, Zhu et al.(Reference Zhu, Ruiz De Azua and Feijen75) found that the spatial chocolate distribution in 3D-printed protein bars affected their perceived hardness. Sensory manipulations profoundly affect the dining experience, food intake and satiety(Reference Stribiţcaia, Evans and Gibbons76,Reference Lin, Punpongsanon and Wen77) . Lin et al.(Reference Lin, Punpongsanon and Wen77) used 3D-printed cookies with the same energy content to obtain different chewing times and satiety by modifying the infill pattern and density of the cookies. The ‘between-bites heterogeneity’ in sensory intensity (e.g. sweetness, saltiness, sourness, umami and bitterness) and their synergistic or antagonistic interactions of 3D-printed food products is particularly interesting. For example, 3D food printing can be used to reduce sugar content in many food products, with the potential to reducing the overall sugar intake across the population. Kistler et al.(Reference Kistler, Pridal and Bourcet78) found that a heterogeneous sucrose distribution across the outer shell and inner core of 3D-printed food products could increase their sweetness perception by >30 %. Similarly, 3D-printed chocolate layered with different sugar concentrations could reduce sugar usage by 19 % without changing the perceived sweetness or overall liking(Reference Khemacheevakul, Wolodko and Nguyen79).
Controlled nutrient release and gut microbiome modulations using three-dimensional food printing
3D printing is suitable to produce personalised medications with diverse controlled release profiles to achieve optimum therapeutic results(Reference Trenfield, Madla, Basit, Basit and Gaisford80,Reference Lim, Kathuria and Tan81) . Controlled nutrient release from 3D-printed food products can be achieved by (i) varying spatial nutrient distribution(Reference Fahmy, Amann and Dunkel72,Reference Zhu, Ribberink and De Wit82) ; (ii) product matrix design (e.g. polypills by constructing multi-ingredient layers with distinct digestion locations and digestibility)(Reference Chi, Li and Huang83) and (iii) modifying geometric designs (e.g. infill density and patterns, shape, surface area)(Reference Trenfield, Madla, Basit, Basit and Gaisford80,Reference Lim, Kathuria and Tan81) . Together with manipulated sensory perception, the controlled digestibility of 3D-printed food products can impact satiety and food intake(Reference Lin, Punpongsanon and Wen77). The same controlled nutrient release strategy could be used for probiotic delivery, faecal microbiota transplantation, faecal filtrate transplantation and phage therapy(Reference Zmora, Suez and Elinav84). It has been reported for example that optimising the printing structures of several 3D-printed food products containing probiotics results in improved probiotic survival after processing(Reference Zhang, Lou and Schutyser74,Reference Yoha, Anukiruthika and Anila85–Reference Kuo, Clark and Qin87) .
Beyond nutrition: consumer acceptance, cost-effectiveness, food safety, ethics and regulations determine three-dimensional food printing adoption
Similar to many other innovations, the real-world adoption of 3D food printing technology depends on consumer acceptance. Only a few empirical studies have investigated consumer attitudes (including allied health professionals) towards 3D food printing in different settings(Reference Lupton32,Reference Rubinsky66,Reference Manstan and McSweeney88–Reference Tesikova, Jurkova and Dordevic94) , with some recently summarised by Baiano(Reference Baiano5). In general, the studies find that consumers have low awareness and knowledge about 3D food printing, suggesting that consumer acceptance of 3D food printing may be challenging due to unfamiliarity with the technology, while some consumers believe 3D-printed food is ‘unnatural’ and are concerned of its health benefits(Reference Baiano5).
Another aspect relevant to the commercial success of 3D food printing is its economic viability and sustainability that can be provided by cost–benefit analysis(Reference Verma, Kamble and Ganapathy27,Reference Jayaprakash, Paasi and Pennanen95) . Dabbene et al.(Reference Dabbene, Ramundo and Terzi96) discussed and introduced an economic model to adopt 3D food printing in the food sector. Rogers and Srivastava(Reference Rogers and Srivastava97) proposed three potential supply chain models for 3D food printing and discussed their key enablers. However, techno-economics and life-cycle assessments for 3D food printing in different settings (e.g. homes, institutions and industry) are primarily uncharted. In addition, the competitive advantage of 3D food printing to other reshaping approaches (e.g. moulding and piping) has not been thoroughly investigated(Reference Smith, Bryant and Hemsley52).
Lastly, authorities around the world have not set regulations on 3D food printing. There are currently no specific regulations on food safety (e.g. allergy, temperature and hygiene conditions) for food materials used in 3D food printing and printed food products, nor are there established shelf-life guidelines or labelling regulations(Reference Sun, Zhou and Huang1,Reference Baiano5,Reference Zhang, Pandya and McClements49) . Tran(Reference Tran98) comprehensively discussed short-term food safety issues (food poisoning, allergy), long-term health risks (potential eating behaviour changes and resulting changes in health) and labelling issues (adulteration and the unknown long-term effects) surrounding 3D food printing. Moreover, 3D food printing could lead to copyright and intellectual property breaches because digital 3D mesh models can be printed directly, distributed and copied(Reference Verma, Kamble and Ganapathy27,Reference Hou, Kim and Ahn99) . In addition, there could be privacy infringements and the illegal use of digital personal information (e.g. 3D scanning, human face images and other personal data)(Reference Grin and Jones100).
Future perspective
In recent years 3D food printing has received exponential interest from both the public and academics due to its potential for personalised nutrition and novel food product development. Currently, 3D food printing can play an indispensable role in redesigning TMF for people with chewing and swallowing difficulties, nutrition literacy, food upcycling and sensory manipulations. However, further evaluation of its benefits, risks and costs is needed before it becomes a trusted food technology in the marketplace, let alone a household kitchen appliance such as microwave ovens(Reference Wahlqvist101). In particular, the prospect of reduced nutritional quality of 3D-printed foods, and limited substrates and vehicles for 3D food printing technology will be challenging(Reference Cannon and Leitzmann102). Nevertheless, the success of 3D food printing will depend on the extent to which it supplants the existing food processing technologies and meets the needs and expectations of various users (e.g. households, institutions and the food industry). Those assigned the role of nutrition counselling in the health care system will need convincing of the utility, safety, acceptability, affordability and short- to long-term health benefits of 3D-printed foods(Reference Wahlqvist103). In turn, its place in a world of increasing food and nutrition insecurity, on account of climate change, population displacement, and inequity, is likely to become more evident(2).
Financial Support
This work is supported by the Western Australian Future Health Research and Innovation Fund, an initiative of the Western Australian State Government. The salary of J. R. L. is supported by a National Heart Foundation of Australia Future Leader Fellowship (ID: 102817).
Conflicts of Interest
None.
Authorship
L. Z., J. R. L. and J. M. H. conceived the research. L. Z. conducted the literature search and analysed data working together with J. R. L. and J. M. H. L. Z. wrote paper with specific input from M. L. W., M. S., C. P. B. and A. D. (nutrition), A. M. and S. P. (marketing and consumer acceptance), K. H. M. S. and M. J. C. (agriculture and food upcycling) and S. K. J. (food science). All authors provided critical feedback on data interpretation and presentation, read and approved the final manuscript.