Introduction
Rainfed agriculture is the mainstay of rural peoples' livelihood in Mali, engaging almost 70% of Mali's labor force and contributing about 40% to the gross domestic product. The semiarid climatic conditions and the fragile soils of Mali have until now supported production of maize (Zea mays), cotton (Gossypium hirsutum), sorghum (Sorghum bicolor), millet (Pennisetum glaucum), fonio (Digitaria exilis), cowpeas (Vigna unguiculata) and peanuts (Arachis hypogaea) as field crops (Cooper and West, Reference Cooper and West2017). The agricultural system however has been challenged by several factors of which water scarcity, land degradation and loss of ecosystems constitute the main. Unpredictable and unreliable precipitation, reduction in the amount of light rainfall, a delay in the onset of the rainy season and lengthening of periods with no rainfall during the wet season, water runoff and a resulting loss of soil nutrients make rainfed agriculture a risky undertaking throughout much of West Africa (Doraiswamy et al., Reference Doraiswamy, McCarty, Hunt, Yost, Doumba and Franzluebbers2007; Feed the Future, 2012). In addition, droughts both between and within seasons, increasingly erratic rains and shallow formations of sandy loams with gravel soils often overlying impenetrable iron pan or laterite are major constraints to improve agricultural productivity in most west African regions (Ellis-Jones et al., Reference Ellis-Jones, Larbi, Hoeschle-Zeledon, Dugje, Teli, Buah, Kanton, Kombiok, Kamara and Gyamfi2012).
Documented evidence (Traoré et al., Reference Traoré, Sidibe, Coulibaly and Bayala2017; Birhanu et al., Reference Birhanu, Traoré, Gumma, Badolo, Tabo and Whitbread2019) reveal that in southern Mali for example runoff from agricultural fields often reaches 20 to 40% of the total annual rainfall, in spite of gentle slopes (1 to 3%). This important runoff reduces the availability of water for crops, which are sensible to drought, and crop yields are affected. Thus agricultural yields and water productivity (WP) are low, often 10% or less of potential (Traoré et al., Reference Traoré, Gigou, Coulibaly and Doumbia2004). There were several attempts of increasing crop production by increasing production area. However, increasing crop productivity has further been challenged by factors such as rising temperature and absence of storage structures for supplementary irrigation (Andersen et al., Reference Andersen, Dione, Jarosewich-Holder, Jean-Claude and Golitzen2008; Ollenburger et al., Reference Ollenburger, Descheemaeker, Crane, Sanogo and Giller2016). There is a strong need to constantly improve and support emerging crop demand in the region through improving soil fertility and soil water availability during the greater part of the rainy season (Ellis-Jones et al., Reference Ellis-Jones, Larbi, Hoeschle-Zeledon, Dugje, Teli, Buah, Kanton, Kombiok, Kamara and Gyamfi2012; Birhanu et al., Reference Birhanu, Traoré, Gumma, Badolo, Tabo and Whitbread2019). This requires an approach that considers improving crops yield and WP, maintaining the health of the natural ecosystems and understanding the social and economic gains from farm based implemented practices in the smallholder farming community.
Contour bunding (CB) is one technique among the many farm based practices implemented in southern Mali. CB was first introduced in the early nineties by the Institut d'Economie Rurale (IER) in Mali and Centre de Coopération Internationale en Recherche Agronomique pour le Développement (CIRAD) (Birhanu et al., Reference Birhanu, Tabo, Sogoba, Nicolas and Wani2014). Both IER and CIRAD with the participation of small scale farmers promoted a renewed approach of this well-known technique, adapted to the management of individual fields and to agriculture with animal draught. The CB technology gained quick acceptance and adopted by the Malian cotton company Compagnie Malienne de Developpment des Textiles (CMDT) to increase the productivity of cotton in southern Mali (Birhanu, Reference Birhanu2015). With the CMDT intervention a total of 2562 cotton producing villages benefited from CB until 2000. The support from CMDT discontinued in the year 2000, and since then individual farmers have taken own initiative to implement CB practices in their fields (Birhanu et al., Reference Birhanu, Traoré, Gumma, Badolo, Tabo and Whitbread2019). Previously few studies (Gigou et al., Reference Gigou, Giraudy, Doucoure, Healy, Traore and Guindo2004, Reference Gigou, Traoré, Giraudy, Coulibaly, Sogoba and Doumbia2006; Traoré et al., Reference Traoré, Sidibe, Coulibaly and Bayala2017) reported the usefulness of CB on crop yield performance and soil water conservation in specific farm level settings. Evidence is lacking on the usefulness of CB in different agro-ecologies on system components that include productivity, environment and socio-economic interactions. Therefore, the objective of this paper is demonstrating the impact of CB technique on system components that include crops and WP, environmental gains and social and economic outcomes in two different agro-ecologies of southern Mali.
Materials and methods
The methodology adopted in the study involved biophysical characterization of the study site and implementation of suitable land and water management technology that is preferred by rural communities and documenting its impact. Two on-farm experimental stations were established in Bougouni district (Flola village) and Koutiala district (M'Pessoba) village for agronomic and environmental monitoring. Agronomic field experimentation on crop productivity was conducted to determine grain yield and biomass for sorghum, maize, groundnut and millet for 3 consecutive years (2015 to 2017). Based on crop yield data and the total amount of rainfall during the growing season WP was calculated. Previous surveys conducted to understand environmental benefits of improved land and water management practices in central and southern Mali were reviewed and additional environmental variables like water runoff and erosion data were collected from the experimental stations using methodologies described in recently published papers (Traoré et al., Reference Traoré, Sidibe, Coulibaly and Bayala2017; Birhanu et al., Reference Birhanu, Traoré, Gumma, Badolo, Tabo and Whitbread2019). The social and economic outcomes of CB were analyzed using data obtained from the household survey conducted in nine villages.
Description of the study area
The study was conducted in nine villages located in two districts (Bougouni and Koutiala) of the Sikasso region in southern Mali (Fig. 1). The Sikasso region with a 70,280 km2 land area and a population of about 2.63 million (Institut National de la Statistique, 2009) is located in southern Mali and is divided into seven major districts namely: Bougouni, Kadiolo, Kolendieba, Koutiala, Sikasso, Yanfolila and Yorosso. The ecosystem of Sikasso is best defined as a Sudanean savanna (Cooper and West, Reference Cooper and West2017) and agriculture characterized by rainfed, small-scale crop, livestock and integrated crop-livestock/agro-pastoral farming systems dominates the economy (IITA, 2016). The Sikasso region receives higher amounts of rainfall in the range of 800 to 1200 mm and considered as the breadbasket of Mali (Ebi et al., Reference Ebi, Padgham, Doumbia, Kergna, Smith, Butt and McCarl2011). Despite this title, 34% of the Mali's poor and 45% of the Mali food poor live in the region (Dury and Bocoum, Reference Dury and Bocoum2012; Eozenou et al., Reference Eozenou, Madani and Swinkels2013).

Fig. 1. Study area.
Figure 2 presents the long term (1970–2018) seasonal rainfall and temperature patterns in the two studied districts, with months of June to September as the main rainfall season contributing 80% of the mean annual rainfall in each district (Table 1). The long term (1970–2018) mean annual rainfall of Bougouni district is 1060 mm, and that of Koutiala is 862 mm. The long term (1970–2018) average monthly maximum and minimum air temperatures in the two districts are 33 & 22°C in Bougouni and 34 & 23°C in Koutiala respectively. The four villages that constitute the study area in Bougouni district are Dieba, Flola, Madina and Sibirila. In Koutiala district the studied villages include M'Pessoba, Nampossela, N'Golonianasso, Sirakélé and Zanzoni (Fig. 1). The geographic coordinates along with population and growing season (June to September) mean annual rainfall over the study villages are presented in Table 1.

Fig. 2. Seasonal variation of rainfall and temperature data in Bougouni and Koutiala districts (data from 1970 to 2018).
Note: RB and RK refer to long term seasonal rainfall in Bougouni and Koutiala districts respectively. Max TB and Max TK refer to long term seasonal maximum temperature in Bougouni and Koutiala respectively. Min TB and Min TK refer to long term seasonal minimum temperature in Bougouni and Koutiala districts respectively.
Table 1. Geographic location, population and mean annual rainfall of the study villages

a Commune is a lower administrative structure next to a district.
b Population data source: Institut National de la Statistique, 2009.
c MAR is the growing season (June to September) rainfall data (averaged from ordinary rain gauges installed in each study village (2015–2017)).
d Insufficient data.
Biophysical (agronomic and environmental) field experimentation
Community participation was vital for monitoring biophysical variables that involve agronomic, water runoff and soil erosion data. Agronomic data on crops yield and biomass, and data on water runoff and erosion were monitored in fields with and without contour bunds. Four water runoff and erosion monitoring stations were established (Fig. 3a and 3b) in each experimental site for water runoff and erosion monitoring. The stations were installed from 1 to 30 June 2015 and data monitoring started immediately. Five rain gauges were installed in each intervention village in both districts.
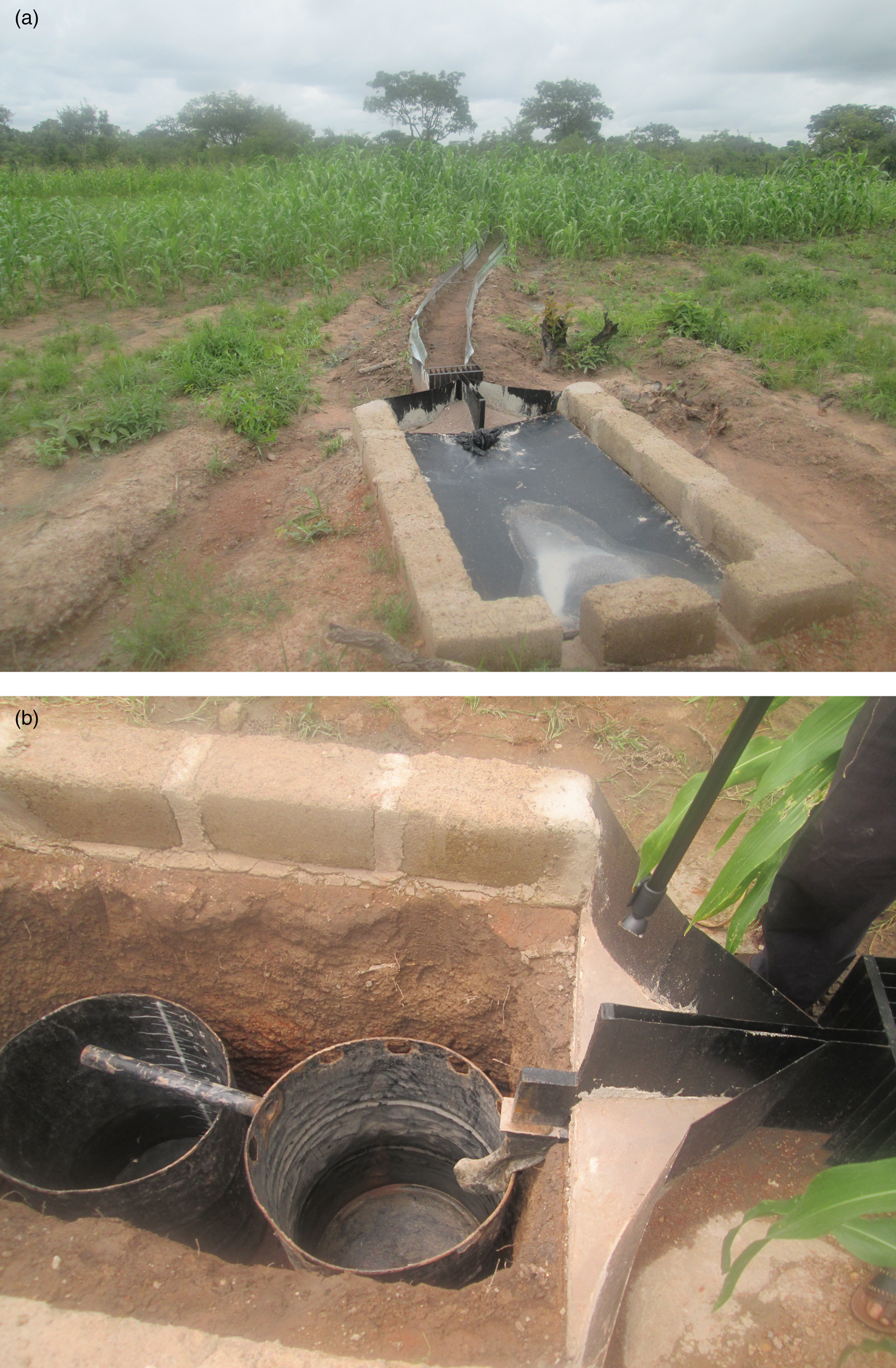
Fig. 3. (a) Structural layout of water runoff and erosion monitoring device and (b) water runoff collection chamber.
The protocol for the design and installation of runoff and erosion monitoring equipment in Flola and M'pessoba experimental sites was obtained from the IER (Birhanu et al., Reference Birhanu, Traoré, Gumma, Badolo, Tabo and Whitbread2019). A runoff and erosion monitoring device was constructed locally. The equipment consists of: (i) an oblique 40 cm long and 33 cm wide iron sheet, (ii) a water supply pipe of 60 cm length, (iii) two tanks of up to 200 liters and (iv) a cover sheet of 180 cm × 100 cm. These were placed in a pit (150 cm × 70 cm × 130 cm) to capture runoff and erosion data from an area of 63 m2 (Fig. 3a and 3b). In each of the experimental sites, water runoff and erosion were monitored in the two adjacent fields. Each field was divided into two parts and four sets of water runoff and erosion equipment were installed. In the first field, ridges followed the CBT while in the second field, traditional farmer practices labelled as non-contour bunding (NCB) were considered as a control. Two replications were implemented in the CB field and two others in the NCB field. The treatment and control plots were homogeneously managed in terms of field operations and crop species. Agronomic data on yield of crops and biomass has been collected in each field and environmental data on water runoff and soil erosion was collected immediately after each rainfall event. The runoff rate (R) was determined for each rainfall event using equations presented below (Equations 1 and 2):


Soil erosion was computed from a product of water runoff and sediment concentration. Runoff water containing sediment samples were taken to the soil plant and water laboratory of IER to determine sediment concentration and nutrient content. Soil chemical properties that were mostly adversely influenced by erosion or topsoil removal include organic carbon content (C), total nitrogen (N), available phosphorus (P), calcium (Ca), magnesium (Mg) and potassium (K) (Mhango et al., Reference Mhango, Snapp and Phiri2013). A total of 40 soil samples were collected from each experimental site and laboratory analysis was done at the soil and plant laboratory of IER to determine nutrient content of the soil. The procedure for runoff water collection, soil sampling protocol and analysis of sediment concentration was conducted according to the methodology described in the recently published work of Traoré and Birhanu (Reference Traoré and Birhanu2019).
Water productivity
WP is defined most often as the average amount of output per unit of water applied on a field (Equation 3) for irrigated systems or per unit of water evapo-transpired (Equation 4) in rainfed systems (Wichelns, Reference Wichelns2014).


According to Cook et al. (Reference Cook, Gichuki and Turral2006) the time period over which WP is estimated is determined by the cycle of agricultural production that drives the system. Normally, this would include at least one complete crop cycle, extended over a complete year to account for productive and non-productive water use.
For agricultural systems the output function (numerator) of Equations 3 or 4 is the primary yield data generated from direct measurement or by crop survey. A key distinction when computing WP is to differentiate between water input to an agricultural system and water depleted by it. WP is estimated from the amount of water directly consumed by the agricultural system (that is, evaporation and transpiration), not simply the amount of water supplied (Cook et al., Reference Cook, Gichuki and Turral2006). For dryland cropping systems however the water consumed (denominator of Equation 4) is simply calculated as:

where Et is the water consumed or evapo-transpired, P Growing season is the total amount of rainfall in a growing season and ΔS is the change in soil moisture in a growing season. In dryland systems, changes in soil water at the beginning and end of growing season may be assumed to be insignificant, so that water consumption is simply estimated as the rainfall amount during the growing season (Cook et al., Reference Cook, Gichuki and Turral2006).
Survey procedure
For the socio-economic aspects, data were collected from a subsample of 45 respondents (27 men, 18 women) from the nine villages in which contour bunds were introduced. Since the sample size restricts the representativeness of the results, we validated our findings against evidence from other research and showed avenues for further investigation. Information collected included: (i) decision-making on the introduction of contour bunds, (ii) labor contributions to the establishment and maintenance of bunds, (iii) the use of draught animals and (iv) the control of income from fields in which contour bunds were established. All data pertaining to these aspects were sex dis-aggregated for gender, allowing the differences in how men and women view such systems to be investigated.
Results
Productivity
From the studied villages the crops maize, sorghum, millet and groundnut accounted for 60% of production. Cotton is the main cash crop with production representing 35% in Bougouni and 29% in Koutiala district (Fig. 4) and reflecting the Compagnie Malienne Pour le Développement des Textiles scheme. Experimental results (Table 2) of CB application on productivity and statistical variations highlighted that the average grain yield of sorghum with the CB plot was 1826 kg ha−1 and 1371 kg ha−1 in a farm without CB (an increase of 33% with P < 0.05). Sorghum biomass increased by 42% in a CB plot (P < 0.05) from 3402 kg ha−1 in a non CB plot (Table 2). For groundnut, the grain yield without CB was 1147 kg ha−1 and an increase of 44% (P < 0.05) was obtained with the use of CB (1661 kg ha−1). A 42% biomass yield advantage (from 1985 kg ha−1) was obtained for groundnut in a CB field as well. Similarly grain yield and biomass of maize increment was obtained for maize by 63 and 71% (P < 0.01) respectively with the use of CB. Grain yield and biomass of millet increased by 78 and 81% (at P < 0.01) with the use of CB. This result is in agreement with a 2 year experimental study (2012 and 2013) with CB at the Cinzana research station in southern Mali that reported the use of CB increased the yield of millet by 72% (Traoré et al., Reference Traoré, Sidibe, Coulibaly and Bayala2017).

Fig. 4. Crop production in Bougouni and Koutiala district.
Table 2. Grain yield of crops in fields with and without CB.

WP refers to water productivity; CB refers to contour bunding; NCB refers to no contour bunding.
*Statistically significant at P < 0.05, **Statistically significant at P < 0.01.
WP of the four crops were also presented in Table 2 using the mean annual rainfall (MAR) of 611 mm for Bougouni (Flola experimental station) and 541 mm for Koutiala (M'Pessoba experimental station) (data presented in Table 1). Observations on WP highlighted that WP is higher in crops grown in CB fields. In addition, in Koutiala WP was found to be higher than that of Bougouni. Wichelns (Reference Wichelns2014) highlighted that estimates of WP provide limited insight regarding farm-level crop production in rainfed and irrigated settings. Higher estimates of WP are not necessarily associated with higher yields or larger amounts of production for sale or home consumption. However, estimates of WP enhance understanding of farm level water management (Wichelns, Reference Wichelns2014) and in depth research that integrates risk, uncertainty, prices, opportunity costs of farm level benefits need to be studied further.
Environmental aspects
After each rainfall event data collected on water runoff showed higher values (more than 45%) in non-treated fields than the CB fields. The magnitude of runoff in non-treated field was more than 100% during the heavy rainy seasons of July and August. The runoff coefficient was significantly higher (P < 0.01) in non-treated fields (ranging from 35 to 39%) to 17 to 21% in CB fields. Data records for 2 years (2016 to 2017) showed that in treatment fields, on average, 162 mm of rainfall per year was saved as soil water and soil loss was reduced by 163% (P < 0.01). On average 13,090 kg ha−1 of soil was lost from farm fields without CB and CB implementation significantly reduced the soil loss (Table 3).
Table 3. Runoff coefficient and soil loss in Bougouni and Koutiala districts during 2016 and 2017 cropping season

Note: values with different letters are statistically different at P = 0.05. Column means represent runoff coefficient and soil loss; row values show technology, experimental site and year of data record.
When the magnitude of rainfall was higher as in the case of Bougouni (with a mean annual rainfall of 1060 mm) than Koutiala (with a mean annual rainfall of 862 mm) the corresponding volume of soil loss was higher by 98% (P < 0.01). Soil loss varied among the years (P < 0.05) with the highest values observed in 2016 (+92%) which had 24% more rain than the year 2017 (Table 3).
Nutrient losses in CB and non CB plots showed significant differences with higher nutrient losses recorded in farms without CB. Losses of organic carbon, nitrogen and phosphors were at least two times higher in non CB fields. Losses of calcium, magnesium and potassium in the non CB plots, were 80, 66 and 75% higher than that of CB plots respectively (Table 4). Results further indicated that there are no distinctive nutrient losses between the two districts (P > 0.05) signifying comparable soil texture and rate of degradation. Except for phosphorous (P = 0.12), nutrient loss was significantly higher in 2016 than 2017 (Table 4). Nutrient losses were recorded on a farm land whose slope is described relatively as flat (1.5 to 2%) in both districts. The soil characteristics of the sites are described as similar in terms of granulometric soil composition. It is obvious that nutrient losses would be higher than the current recorded values in farmlands located at higher slope areas and non-treated with CB. It is therefore important to expand the study of CB in a wider heterogeneous landscape and record the benefits.
Table 4. Nutrient losses in eroded soil (kg ha−1 yr−1) under CB and non CB in Bougouni and Koutiala districts during 2016 and 2017 cropping season

Note: values with different letters are statistically different at P = 0.05.
Social aspects
In the social domain, we investigated household decision-making on the introduction of contour bunds, labor contributions and the use of draught animals to establish and maintain bunds. A total of 89% of the respondents from male-headed households indicated that the head had made the decision to establish contour bunds. In five out of the seven female-headed households, the female head had opted for the technology, while in the remaining two a male spouse who was not currently living on the farm had made the decision to establish CB. Prominent male decision-making on technology and land use is confirmed by other studies in the Malian context (Grosz-Ngaté, Reference Grosz-Ngaté, Bingen, Robinson and Staatz2000; Wooten, Reference Wooten and Howard2003; Doka et al., Reference Doka, Madougou and Diouf2014).
Respondents indicated that contour bunds, for the most part, were established and maintained by young men or adult male household members along with limited labor contributions by women (Table 5). This is contrary to other findings, which describe married women (but not unmarried youth) as important part of the agricultural labor force (Grosz-Ngaté, Reference Grosz-Ngaté, Bingen, Robinson and Staatz2000; Doka et al., Reference Doka, Madougou and Diouf2014). Three reasons could explain the discrepancy: first, 93% of the households in our sample employ draught animals for the construction of bunds, animals predominantly being handled by men. In male-headed households, women did not own draught animals and joint ownership was negligible (1%; for similar results refer to de Groote and Coulibaly, Reference De Groote and Coulibaly1998). However, six out of the seven women heads were in possession of draught animals. Second, we focused on one labor step only. Subsequent investigations should capture more labor steps (including access to various implements) to better assess the overall contribution of men and women in the cultivation process. Third, contour bunds seem to be established mainly for staple crops. In the socio-cultural contexts of this study, men are responsible for the provision of staple crops to the household (including labor in respective fields), while women often focus on the cultivation of vegetables (Wooten, Reference Wooten and Howard2003).
Table 5. Labor contribution to the establishment and maintenance of contour bunds

Economic aspects
In terms of the control of income from crop sales, in male-headed households the head controlled income from maize (79%), followed by groundnut (77%), cotton (74%), millet (63%) and sorghum (58%). Groundnut and cotton are cultivated as cash crops. Overall, women in male-headed households had less control over income than men. Women in female-headed households indicated a high level of decision-making power with regard to income. Interestingly, the three crops men heads controlled the strongest (maize, cotton and groundnut), were the crops respondents perceived as having the highest income increase after the introduction of contour bunds. Further studies should more clearly distinguish between income from household fields (often controlled by the head) and income from individually cultivated fields with individual income control (see de Groote and Coulibaly, Reference De Groote and Coulibaly1998). It should be noted that women's individual income is often threatened by the following gender dynamics in the study context: Men have a high level of land ownership and control. This results in insecure land tenure for women's individually cultivated fields and the danger that fields women have rehabilitated (for instance through contour bunds) are appropriated by male relatives, as has happened in some cases during the intervention investigated in this paper (refer Doka et al., Reference Doka, Madougou and Diouf2014 for similar processes). The findings for the social and economic domains show gender imbalances that future agricultural interventions should consider and act against, for example through household methodologies (Bishop-Sambrook and Farnworth, Reference Bishop-Sambrook and Farnworth2014).
Discussion
Most of the arable lands in Mali are degraded and prone to heavy erosion due to rain downpours and other factors such as deforestation and population growth (Traoré and Birhanu, Reference Traoré and Birhanu2019). With the application of CB practices in their fields farmers saw that soil and water were conserved, soil erosion and gulley formation was reduced and fertility of the soil has been improved. The ridges of CB increased infiltration of rainwater by up to 10% of the total annual rainfall (960 mm) with one to two percent slopes (Traoré et al., Reference Traoré, Gigou, Coulibaly and Doumbia2004). Recent studies (Traoré et al., Reference Traoré, Sidibe, Coulibaly and Bayala2017; Birhanu et al., Reference Birhanu, Traoré, Gumma, Badolo, Tabo and Whitbread2019) also confirm the use of CB significantly reduces runoff from farm fields and reduce erosion by 73%. The result of the present study showed improvements in a small experimental sites (farm fields) that are characterized with an average slopes of 1.5 to 2% and with similar soil texture (Traoré and Birhanu, Reference Traoré and Birhanu2019). It is recommended to evaluate the application of CB across a wider heterogeneous landscape to understand the benefits.
The experimental results in crop productivity showed that implementation of CB in farmers' field provided higher yield increase mainly for millet and maize crops than sorghum. Apart from improvements in soil water and nutrient retention, genetic variety of crops used for experimentation and suitability of soil for the type of crops grown could be factors for higher yield gains for millet and maize in CB fields. In an area where the soil is dominated by sandy loam soil, millet is more resilient to soil water variations than sorghum and a better yield could be obtained for millet than sorghum. Maize production is more favorable in areas with better rainfall record (Bougouni district) and improved presence of soil water as in the case of CB fields. The choice of right planting dates, presence of adequate soil moisture over an extended period during the crop physiological growth result in variations in yield gains by different crops. However, this concept requires crop physiological study and further agronomic research is recommended.
Findings from the social and economic investigation show that farmers perceive to gain higher incomes from CB fields than from those cultivated under conventional practice. Men predominantly control these incomes. Also, a high labor involvement of men in the application of the technology was reported. However, a broader investigation (combining quantitative and qualitative methods) should shed more light on women's opportunities and constraints to practice soil and water management and benefit from it. This would demand more attention to women's access to land, their tenure security and their room to generate own income from rehabilitated fields. Women's lack of decision-making power on land constitutes a barrier for the adoption of the technology on their individually managed fields as well as on household fields. Furthermore, if women want to establish CB with draught animals on their individual fields, they depend on men for labor support. Therefore, a transformation of underlying inequitable norms and rules is needed to promote climate-smart technologies and to ensure equitable outcomes.
The findings presented in this study conforms with results presented by Chai et al. (Reference Chai, Gan, Turner, Zhang, Yang, Niu, Siddique and Sparks2014) who reported positive interaction among productivity, social, economic and environmental components with the application of CB in the arid and semiarid northwestern China. In Nepal, system interactions with the application of CB have also been reported and smallholder farming communities used CB to control soil erosion, promote water retention and increase crop production (Regmi et al., Reference Regmi, Aryal, Subedi, Shrestha and Tamang2001).
Interactions between components of sustainability that include productivity, environment, social and economic aspects (Pretty, Reference Pretty1997; Laris et al., Reference Laris, Jeremy, Foltz and Voorhees2015) provide important avenues for future research. Especially trade-offs between soil and water retention and potentially increased labor merit a closer investigation. Land preparation with CB may be more labor-intensive than under flat cultivation. It remains open whether the initial high labor investment is offset in later labor steps (for instance through a decreased need for weeding). A comparison between the drudgery involved in various labor steps under CB and under flat cultivation could yield further insights. Another potential trade-off relates to the question whether livestock trample bunds in the dry season thereby making maintenance more difficult. At this point conflicts between livestock keepers and cultivators could be fuelled. All in all, to facilitate climate change adaptation in a sustainable manner (Vanlauwe et al., Reference Vanlauwe, Coyne, Gockowski, Hauser, Huising, Masso, Nziguheba, Schut and Van Asten2014; Stevenson et al., Reference Stevenson, Vanlauwe, Macoursch, Johnsona, Krishnana, Place, Spielman and Vlekg2019), investigations and interventions have to broaden their scope to take account of questions related to productivity, environment and the social and economic domains and their interaction. With regard to barriers of adoption, particularly from the perspective of gender and labor, there is a need to continuously sensitize the farming community on better and adaptable climate change practices (Rao et al., Reference Rao, Gopinath, Prasad, Prasannakumar, Singh and Sparks2016; Regmi et al., Reference Regmi, Aryal, Subedi, Shrestha and Tamang2001).
Conclusions and recommendations
Low agricultural productivity and poor soil fertility in part due to land degradation have been threatening the livelihoods of rural Malians since decades. The increasing population pressure on the natural eco-system and variability of the erratic rainfall call for action to reverse the poor living conditions. The study highlighted the benefit of implementing CB on farmer's fields to improve crop productivity, increase gains in environmental and socio-economic outcomes. Though experimental results are limited to two agro-ecologies in southern Mali, the information helped to increase awareness to land managers, decision makers and farmers on the existing threats related to land degradation and the potential benefits of farm level intervention practices like that of CB. To improve the wider application of CB in rural Mali, awareness campaign and special support programs need to be provided to rural smallholder farming communities to increase landscape wide application of CB.
Acknowledgement
This work was supported by the CGIAR program on Water, Land and Ecosystem (WLE) and the Africa Research in Sustainable Intensification for the Next Generation (Africa RISING) project in Mali. The authors are very grateful to the financial support provided by the International Water Management Institute (IWMI) and the United States Agency for Development (USAID) through the International Institute of Tropical Agriculture (IITA). We are also thankful to the two anonymous reviewers whose constructive comments and suggestions improved the scientific content of the study. The rural communities of Bougouni and Koutiala in southern Mali deserve special thanks and the engagement of the two local NGOs, AMEDD and FENABE during the initial stages of data collection is greatly appreciated.