- DR
-
Dietary restriction
- AL
-
ad libitum
- WNPRC
-
Wisconsin National Primate Research Centre
- NIA
-
National Institute of Aging
- HFD
-
high-fat diet
- TOR
-
target of rapamycin
Caloric restriction is defined as a reduction in energy intake relative to that consumed normally by individuals with free (ad libitum (AL)) access to food, while maintaining nutrient intake( Reference Trepanowski, Canale and Marshall 1 ). For the purposes of this review, the term dietary restriction (DR) will be used exclusively. In this context, DR will include both caloric restriction, as defined earlier, but will also encompass those interventions in which specific macro/micronutrients (e.g. protein, carbohydrate and amino acids) are restricted without any reduction in energy intake per se ( Reference Trepanowski, Canale and Marshall 1 – Reference Shimokawa, Higami and Yu 4 ). While the periods of fasting undertaken by religious ascetics have been known for centuries to increase awareness and activity( Reference Mehta and Roth 5 ), the first experimental evidence that a restricted diet could impact positively on lifespan was provided by Osborne et al. almost 100 years ago( Reference Osborne, Mendel and Ferry 6 ). These authors reported that DR caused stunting of female rats, resulting in extended lifespan and a longer reproductive period, relative to non-stunted controls. Approximately 10 years later, McCay et al. reported that lifespan could be extended in brook trout (Salvelinus fontinalis) fed a low-protein diet( Reference McCay, Bing and Dilley 7 ), and then went on to generate a significant body of work over several decades examining how DR impacted on lifespan primarily in white rats (reviewed in( Reference Swindell 8 )). Their initial observations showed that lifespan of male rats was increased by DR when initiated immediately after weaning or from 2 weeks post-weaning( Reference McCay, Crowell and Maynard 9 ). While they initially detected no effect in female rats( Reference McCay, Crowell and Maynard 9 ), later studies did find significant DR-induced lifespan extension across both genders( Reference McCay, Sperling and Barnes 10 ). McCay et al. also provided the first tangible evidence that DR could benefit health, as several chronic diseases, including cancer, were delayed and/or decreased in incidence relative to AL controls( Reference McCay, Sperling and Barnes 10 ).
The universality of dietary restriction on lifespan and healthspan
Since the findings of Osborne et al.( Reference Osborne, Mendel and Ferry 6 ), a huge body of work has examined DR across many species, primarily in the quest to unravel the mechanisms driving the ageing process. This research clearly demonstrates that DR is the most reproducible environmental intervention through which median and maximum lifespan can be extended( Reference Weindruch and Walford 11 – Reference Masoro 16 ). The positive effects of DR on lifespan have been reported in model organisms such as yeast (Saccharomyces cervisiae), nematodes (Caenorhabditis elegans), fruit flies (Drosophila sp.), mice and rats( Reference Weindruch and Walford 11 ), but also in non-model organisms, including spiders( Reference Austad 17 ), Hereford cattle( Reference Pinney, Stephens and Pope 18 ) and Labrador dogs( Reference Kealy, Lawler and Ballam 19 ). This has led to the widely held belief that DR effects on longevity are universal, although, as discussed later in this review, recent findings suggest that this is not the case.
As reported by McCay et al.( Reference McCay, Sperling and Barnes 10 ) in their early studies and substantiated subsequently in many other studies is that DR postpones and/or ameliorates a wide range of both age-related and non-age-related pathologies (for discussion see( Reference Weindruch and Walford 11 , Reference Speakman and Mitchell 14 )). Rodents under DR are significantly less susceptible to spontaneous cancers, showing a delay in the initiation and an attenuation in the progression of various tumours( Reference Weindruch and Walford 11 ). Similarly, the growth of transplanted and induced tumours is slowed by DR( Reference Kalaany and Sabatini 20 , Reference Giovanella, Shepard and Stehlin 21 ). DR rodents are also significantly leaner than AL animals, and present a broad-spectrum attenuation in many age-associated pathologies including insulin resistance, glucose intolerance, immune dysfunction, cognitive decline, sarcopaenia and cataracts( Reference Weindruch and Walford 11 – Reference Speakman and Mitchell 14 , Reference Masoro, Yu and Bertrand 22 – Reference Selman, Phillips and Staib 24 ). DR also enhances stem cell function in skeletal muscle( Reference Cerletti, Jang and Finley 25 ), and reduces pathology in mouse disease models, including Alzheimer's disease( Reference Halagappa, Guo and Pearson 26 ), pancreatic cancer( 27 ), amyotropic lateral sclerosis( Reference Miller, Robinson and Reuland 28 ) and viral myocarditis( Reference Kanda, Saegusa and Takahashi 29 ).
While the effects of DR on lifespan in rhesus monkeys (Macaca mulatta) are currently unclear (see the following and references therein), DR does confer many health benefits in these animals( Reference Colman, Anderson and Johnson 30 , Reference Chen, Liu and Zheng 31 ). Rhesus monkeys on DR enjoy a greater period of their life free from several age-related diseases including type 2 diabetes, cancer and CVD (( Reference Colman, Anderson and Johnson 30 ), but see also( Reference Chen, Liu and Zheng 31 )). DR also protects rhesus monkeys against sarcopaenia, adiposity, brain atrophy, heightened stress-associated behaviours and significantly reduces plasma TAG and fasting glucose levels( Reference Colman, Anderson and Johnson 30 – Reference Rera, Bahadorani and Cho 35 ). However, as in rodents, it appears that ultimately those diseases that cause death in AL and DR monkeys are somewhat similar, that is DR compresses the period of morbidity suffered in old age( Reference Chen, Liu and Zheng 31 ).
Evidence against the universality of dietary restriction on lifespan
Although significant conservation exists in the beneficial effects of DR on lifespan across evolutionary distant organisms, several studies report DR having no effect, or indeed a negative effect, on lifespan( Reference Chen, Liu and Zheng 31 , Reference Carey, Liedo and Harshman 36 – Reference Sohal, Ferguson and Sohal 41 ). The precise reasons as to why some species or genetic strains within a species respond differently to DR is currently unclear, but there is great current interest in using comparative approaches to examine this( Reference Austad 42 – Reference Selman, Blount and Nussey 44 ). In mice, at least, the genetic background appears to be an important factor in the DR response with the DBA/2 mouse being a case in point. Although a debate exists on whether this strain actually responds positively( Reference Turturro, Witt and Lewis 45 ) or negatively to DR( Reference Fernandes, Yunis and Good 46 , Reference Forster, Morris and Sohal 47 ), several studies have employed this strain as a ‘negative’ responder( Reference Sohal, Ferguson and Sohal 41 , Reference Ferguson, Sohal and Forster 48 – Reference Li, Rebrin and Forster 50 ). While the effects of DR on the lifespan in DBA/2 mice are ambiguous, when a positive effect on lifespan has been reported it tends to be more moderate compared with strains such as C57BL/6( Reference Turturro, Witt and Lewis 45 ). It is also clear that these two strains show significant differences in physiology both under AL and the DR diets. For example, under AL conditions, the DBA/2 mice are relatively more hyperinsulinaemic, insulin resistant and fatter than C57BL/6 mice, with reduced bone mineral density and higher mass-specific resting metabolic rates( Reference Sohal, Ferguson and Sohal 41 , Reference Hempenstall, Picchio and Mitchell 51 – Reference Muzumdar, Allison and Huffman 55 ). In addition, the improvement in glucose tolerance seen following short-term DR is significantly slower in DBA/2 mice than in C57BL/6 mice( Reference Hempenstall, Picchio and Mitchell 51 ).
In ageing studies in mice the strain of choice has been C57BL/6, although even here significant inter-study differences exist in how DR affects lifespan. On using a meta-analysis-type approach to examine twenty-two separate DR experiments in C57BL/6 mice, the effect of DR on median lifespan ranged from a 26·8% increase to a 32·8% decrease, relative to AL controls( Reference Swindell 8 ). Indeed, the average increase across all the studies was only 6·7%( Reference Swindell 8 ). There are many potential reasons as to why disparity exists across the studies. Differences in animal husbandry and housing (e.g. health status and diet) and experimental design (e.g. age at initiation of DR, level of DR and gender) may all effect on the level of lifespan extension reported( Reference Swindell 8 , Reference Barger, Walford and Weindruch 13 , Reference Selman, Lingard and Gems 56 , Reference Liang, Masoro and Nelson 57 ). For example, C57BL/6 mice appear sensitive to both the age at which DR is initiated and to exactly how DR is introduced( Reference Swindell 8 ), i.e. full DR started immediately or a step-down regimen to full DR( Reference Miller, Chang and Galecki 58 , Reference Hempenstall, Page and Wallen 59 ). In mice, the later DR is initiated in the lifespan continuum the smaller the magnitude of lifespan extension( Reference Weindruch and Walford 60 – Reference Speakman and Hambly 62 ), with some studies reporting no effect( Reference Lipman, Smith and Bronson 63 ) or a negative effect( Reference Forster, Morris and Sohal 47 ) when DR is started in middle-age or late in life. The absence of significant lifespan effects in some studies may be due to small sample sizes, whereby single individuals have a large effect on the overall group survival( Reference Barger, Walford and Weindruch 13 , Reference Liang, Masoro and Nelson 57 ). Indeed, and as reviewed in detail elsewhere( Reference Swindell 8 ), contemporary analysis of some of the early rat DR studies suggest that the lifespan extension effects reported may have been somewhat overestimated. Large sample sizes may also produce negligible but significant lifespan effects that might have little relevance to the actual biology( Reference Swindell 8 ). There are also some suggestions that DR may affect the ageing trajectory differently in mice and rats, and this may also be the case within different strains. For example, demographic studies have suggested that in rats the rate of ageing is retarded following DR but that the onset of ageing (initial mortality rate) is delayed in mice( Reference Gan, Hu and Jiang 64 ), although other studies report that the ageing rate is retarded in both( Reference Simons, Koch and Verhulst 65 ). Another popular notion is that DR extends median lifespan by a similar extent to the level of restriction imposed, i.e. 30% DR will increase lifespan by ∼30%, 40% DR will increase lifespan by ∼40% and so on to an upper limit whereby starvation is imposed. Swindell( Reference Swindell 8 ) recently examined 125 rodent DR lifespan studies (fifty-three rat/seventy-two mouse) run between 1934 and 2012 and reported that the increases in lifespan following DR are much more conservative, particularly in mice. DR increased rat median lifespan by 30% and maximum lifespan by 32%, but in mice the increase was only 15% and 18% for median and maximum lifespan, respectively( Reference Swindell 8 ). Indeed, a quarter of rat studies showed an increase in median lifespan of 13·8%, whereas in mice a similar analysis uncovered an increase of only 4·1%( Reference Swindell 8 ). This same study also reported contrasting gender effects, with males rats, but female mice, showing the biggest effects of DR on lifespan( Reference Swindell 8 ).
The impact of dietary restriction on lifespan in ILSXISS recombinant inbred mice
The relevance of genetic background to the DR response was recently demonstrated by two studies undertaken at the University of Texas( Reference Liao, Rikke and Johnson 66 ) and the University of Colorado( Reference Rikke, Liao and McQueen 67 ). Both studies used an unbiased screening approach to examine lifespan in ILSXISS recombinant inbred mouse strains; a heterogeneous stock derived from eight distinct mouse strains used initially to examine ethanol sensitivity( Reference Williams, Bennett and Lu 68 ). In the first study( Reference Liao, Rikke and Johnson 66 ), lifespan in male (forty-one strains) and female (thirty-nine strains) mice was assayed following exposure to 40% DR initiated between ages ∼60 and 150 d. Surprisingly, DR reduced lifespan in more strains than it increased. Indeed, only 5% of male (Fig. 1a) and 21% of female (Fig. 1b) strains showed a significant lifespan extension under DR, with more (27% and 26% for males and females, respectively) showing a reduction in lifespan relative to AL controls. In the second study( Reference Rikke, Liao and McQueen 67 ), females from forty-two different strains under 40% DR were studied. DR was initiated between ages 28 and 40 d, and included twenty-nine strains also used in the earlier study( Reference Liao, Rikke and Johnson 66 ). A significant increase in lifespan following DR was reported in 21% of strains (Fig. 1c), with a similar number (19%) showing a significant shortening of lifespan following DR( Reference Rikke, Liao and McQueen 67 ). No association was detected between AL and DR mean lifespan, implying that different processes may modulate lifespan under different feeding conditions, although maximum lifespan did correlate( Reference Rikke, Liao and McQueen 67 ). The factors leading to these strain-specific responses are unclear, but lifespan extension under DR was inversely correlated with fat loss( Reference Liao, Rikke and Johnson 69 ). A recent reanalysis of this data indicates that when the positive and negative responders are separated, the negative relationship between lifespan and fat mass is detected only in the negative responders( Reference Speakman and Mitchell 14 ). This implies that losing fat mass may not be central to lifespan extension but that DR may push the negative responders past a point whereby DR is beneficial due to energy imbalance( Reference Speakman and Mitchell 14 ). What is clear is that the ILSXISS inbred strains are likely to be invaluable in attempting to disentangle the precise molecular mechanisms underlying DR. The number of animals used in these studies ranged between five and six individuals per group, which may, as discussed earlier, invite some criticism that single animals may have had large effects on overall group survival( Reference Barger, Walford and Weindruch 13 , Reference Liang, Masoro and Nelson 57 ), although this is pretty much unavoidable in these large-scale screening experiments. It is also currently unclear as to whether the separate strains on DR (or AL) died of different things. However, as the authors themselves allude to the critical next steps will be to repeat the DR studies using larger sample sizes, examine strain-specific responses to different grades of DR and examine cause of mortality across the different strains( Reference Speakman and Mitchell 14 , Reference Liao, Rikke and Johnson 66 ).
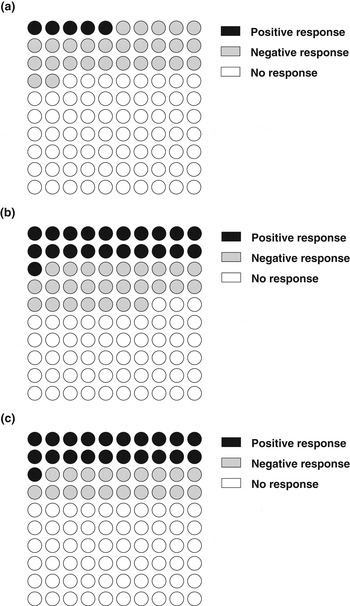
Fig. 1. The percentage of ILSXISS strains from the studies of Liao et al.( Reference Liao, Rikke and Johnson 66 ) and Rikke et al.( Reference Rikke, Liao and McQueen 67 ) demonstrating significant positive (black), significant negative (grey) or non-significant (unfilled) effects of 40% DR on mean lifespan (relative to AL controls; significance P < 0·05). A total of forty-one male (a) and thirty-nine female (b) strains were studied by Liao et al.( Reference Liao, Rikke and Johnson 66 ), with forty-two female (c) strains studied by Rikke et al.( Reference Rikke, Liao and McQueen 67 ); n 5 and 5–6 per strain for( Reference Liao, Rikke and Johnson 66 ) and( Reference Rikke, Liao and McQueen 67 ), respectively. Each dot represents 1% of total.
Dietary restriction in non-human primates
As discussed earlier, the beneficial impact of DR on a wide spectrum of health-related pathologies is apparent in primates, even if the effects on lifespan are less clear( Reference Colman, Anderson and Johnson 30 , Reference Chen, Liu and Zheng 31 ). In the earliest study by Colman et al.( Reference Colman, Anderson and Johnson 30 ), run at The Wisconsin National Primate Research Centre (WNPRC), DR had no effect on survival when all-cause mortality was reported. However, the lifespan curves of the two groups appear to be clearly separating, with <50% of the DR animals actually dead at the time this research was published. However, when non-ageing related causes of mortality were excluded from the survival analysis (gastric bloat, endometriosis, injury and anaesthesia), a statistically significant increase in lifespan was detected in the DR cohort. This suggests that primates under DR are more prone to non-age-related causes of mortality. In the second study undertaken by the National Institute of Aging (NIA), DR initiated in early- or late-life had no beneficial effect on lifespan for all-cause mortality or for age-related mortality (P = 0·06) in the young-onset cohort( Reference Chen, Liu and Zheng 31 ). Again, a completed mortality data set for the young-onset group is unlikely to be completed for at least 10–15 years from now, but unlike the WNPRC study, there does not appear to be any clear inkling that lifespan will be extended in the DR animals upon completion.
It is important to note that both studies differed in several aspects of the experimental design and husbandry that may explain some of the differences reported( Reference Chen, Liu and Zheng 31 , Reference Partridge 70 ). In the WNPRC study, the control animals had no set limit to their daily food intake( Reference Colman, Anderson and Johnson 30 ), whereas food intake of controls in the NIA study was regulated to help avoid obesity, resulting in slight DR( Reference Chen, Liu and Zheng 31 ). Moderate DR (8%) can delay age-related changes in skeletal muscle in rats for example( Reference Kim, Kwak and Leeuwenburgh 71 ). Therefore, the lack of effect of DR on lifespan in the NIA study may, as they themselves suggest, potentially be because the additional benefits of full DR on lifespan was minimal relative to those provided by moderate DR in the controls( Reference Chen, Liu and Zheng 31 ). Further variability between the two experimental designs included diets with different dietary composition (e.g. 28·5% sucrose in WNPRC and 3·9% in NIA), differences in mineral and vitamin supplementation given to the DR animals, distinct countries of origin of the animals which impacted on genetic diversity between experimental cohorts, and also differences in the age of onset of DR( Reference Colman, Anderson and Johnson 30 , Reference Chen, Liu and Zheng 31 , Reference Partridge 70 , Reference Austad 72 ). One final difference between the studies was that the WNPRC employed a medical intervention policy while the NIA originally did not, although they did subsequently intervene to treat endometriosis( Reference Chen, Liu and Zheng 31 ).
Is dietary restriction a realistic intervention in human subjects?
The lack of any clear effect on lifespan in the non-human primate studies is perhaps slightly sobering for proponents of DR in human subjects. Certainly the question of whether DR is capable of extending lifespan in human subjects is a continued source of debate( Reference Speakman and Mitchell 14 , Reference Speakman and Hambly 62 , Reference Phelan and Rose 73 – Reference Cava and Fontana 75 ), although this is unlikely to be answered any time soon given that randomised control trials tracking human lifespan are improbable. In rodents the later in life DR is started then the more modest the effects on lifespan are( Reference Weindruch and Walford 60 – Reference Speakman and Hambly 62 ), with extrapolations from rodent studies suggesting that an individual commencing on DR at ∼48 years, will only add an additional 2·8 years to their average lifespan of 78 years( Reference Speakman and Hambly 62 ). However, what is unequivocal is that DR induces significant health benefits in many animals, and arguably what is really important is a greater period of total life free from age-related disease rather than an extension of lifespan per se. In human subjects, it is evident that even short-term and relatively moderate DR in overweight and obese individuals, and in non-obese individuals, can confer a multitude of health benefits( Reference Cava and Fontana 75 – Reference Larson-Meyer, Heilbronn and Redman 78 ). For example, DR appears to protect against a range of age-related diseases in human subjects, including obesity, CVD, arteriosclerosis and hypertension( Reference Cava and Fontana 75 – Reference Weiss, Racette and Villareal 81 ). DR also slows age-related declines in left ventricular diastolic function, increases insulin sensitivity and reduces the levels of several inflammatory cytokines( Reference Cava and Fontana 75 ). It also can slow the progression of sarcopaenia, possibly through the induction of skeletal muscle mitochondrial biogenesis and a reduction in muscle DNA damage( Reference Civitarese, Carling and Heilbronn 82 ).
Dietary restriction mimetics
It is clear that despite the significant beneficial effects of DR on multiple markers of health in human subjects, the reality is that life-long DR is unlikely to be a realistic or popular life choice for most of the human population. Consequently, substantial research effort is currently being focused on identifying DR mimetics, i.e. compounds that can capture the benefits of DR without the requirement for chronic food restriction. A whole host of putative DR mimetics have been reviewed in detail elsewhere( Reference Dhahbi, Mote and Fahy 83 – Reference Spindler 86 ), but this review will concentrate on three compounds currently enjoying much of the limelight as plausible DR mimetics; rapamycin, metformin and resveratrol.
Rapamycin
Rapamycin is a microlide drug originally isolated from soil-derived bacteria on Easter Island. It has extensive clinical use as an immunosuppressant and as a treatment for various cancers, acting primarily to inhibit the nutrient-sensing target of rapamycin (TOR), a kinase complex involved in growth and metabolism( Reference Lamming, Ye and Astle 87 – Reference Lamming and Sabatini 89 ). The TOR kinase in mammals (mTOR) acts as the catalytic subunit in two distinct complexes, mTORC1 and mTORC2( Reference Lamming and Sabatini 89 , Reference Lamming, Ye and Sabatini 90 ). Originally it was suspected that rapamycin only inhibited mTORC1, although more recently chronic rapamycin treatment has also been shown to inhibit mTORC2( Reference Lamming and Sabatini 89 ). In genetically heterogeneous (HET3) mice, dietary rapamycin treatment initiated either early or late in life significantly extended lifespan in both male and female animals( Reference Miller, Harrison and Astle 91 , Reference Harrison, Strong and Sharp 92 ). Intermittent rapamycin treatment via injections three times per week over a 2-week period every month also increased lifespan in female cancer prone mice( Reference Anisimov, Zabezhinski and Popovich 93 ) and in tumour bearing female 129/Sv mice( Reference Anisimov, Zabezhinski and Popovich 94 ). In adult Drosophila, treatment with rapamycin has been shown to increase lifespan and stress resistance( Reference Bjedov, Toivonen and Kerr 95 ), with genetic modulation of the TOR signalling clearly appearing to be a highly conserved modulator of ageing in multicellular animals( Reference Selman, Tullet and Wieser 96 – Reference Stanfel, Shamieh and Kaeberlein 99 ).
In a recent, comprehensive study of ∼150 molecular, cellular, functional and pathological age-related traits measured in about twenty-five different tissues alongside mortality curves in male C57BL/6 mice, it was shown that rapamycin extended lifespan when initiated at three different ages (4, 13 and 20–22 months)( Reference Neff, Flores-Dominguez and Ryan 100 ). However, rapamycin's effect on the various age-related traits was less clear, appearing to induce many age-independent effects rather than altering ageing per se ( Reference Neff, Flores-Dominguez and Ryan 100 ). In cancer-prone mice, rapamycin treatment has been shown to decrease the average number of mammary adenocarcinomas per mouse and reduced intrinsic tumour size( Reference Anisimov, Zabezhinski and Popovich 93 ). A reduction in spontaneous cancer was also reported in rapamycin-treated mice( Reference Anisimov, Zabezhinski and Popovich 94 ), with the severity of age-related liver degeneration (males), endometrial hyperplasia (females), and incidence of atypical myocardial nuclei and adrenal tumour (both sexes) reduced relative to age-matched controls( Reference Wilkinson, Burmeister and Brooks 101 ). Rapamycin has also been shown to protect aged mice against pneumococcus infection( Reference Miller, Chu and Xie 102 ), and elicits beneficial effects on both dendritic cells and specific stem cell populations( Reference Araki, Ellebedy and Ahmed 103 ) Similarly, long-lived female mice globally null for S6K1, a key downstream effector of mTOR, are long-lived and resistant to several age-related pathologies( Reference Selman, Tullet and Wieser 96 ). S6K1 null mice also show significant transcriptional overlap in liver compared with DR mice( Reference Selman, Tullet and Wieser 96 ), and possess many phenotypic similarities to DR mice( Reference Selman, Tullet and Wieser 96 , Reference Pende, Kozma and Jaquet 104 ).
Rapamycin, however, has several side-effects including immunosuppression in human subjects( Reference Kaeberlein and Kapahi 105 ), although its effects on adaptive and innate immunity appear complex( Reference Araki, Ellebedy and Ahmed 103 , Reference Araki, Turner and Shaffer 106 ). In mice, rapamycin treatment increased testicular degeneration and cataract incidence( Reference Wilkinson, Burmeister and Brooks 101 ), and caused insulin resistance in some mouse strains. In a set of elegant studies, it was recently reported that the longevity effects of DR (via inhibition of mTORC1) can be uncoupled from the insulin-resistant effects (via inhibition of mTORC2( Reference Lamming and Sabatini 89 )). In line with this, life-long insulin resistance is not a pre-requisite for lifespan extension in mice( Reference Selman, Lingard and Choudhury 107 , Reference Selman, Partridge and Withers 108 ). The effects of rapamycin on insulin resistance also appear complex, inducing insulin resistance in C57BL/6 mice( Reference Lamming and Sabatini 89 ) and Sprague–Dawley rats( Reference Houde, Brule and Festuccia 109 ), but not in genetically heterozygous (HET3) mice( Reference Lamming, Ye and Astle 87 ). The duration of rapamycin treatment also appears important in terms of side-effects. For example, in mice a hyperinsulinaemic, insulin resistant and glucose intolerant phenotype was reported in mice after 2 weeks of treatment but this was replaced by a hypoinsulinaemic and insulin sensitive phenotype following 20 weeks of treatment( Reference Fang, Westbrook and Hill 110 ).
Metformin
Metformin is a widely prescribed biguanide antihyperglycaemic drug used to treat type 2 diabetes. It primarily acts to reduce hepatic glucose output, apparently through reducing ATP and cyclic AMP production, suppressing glucagon signalling and activating AMPK( Reference Miller, Chu and Xie 102 , Reference Miller and Birnbaum 111 ). The first evidence that metformin could modulate lifespan was provided by Anisimov et al. almost 10 years ago. They showed that metformin given in drinking water extended mean and maximum lifespan of mammary cancer-prone FVB/N HER-2/neu transgenic mice, and reduced the incidence and size of adenocarcinomas( Reference Anisimov, Berstein and Egormin 112 ). Metformin also reduced the growth of transplanted mammary tumours in FVB/N male mice( Reference Anisimov, Egormin and Piskunova 98 ). However, its effects in inbred 129/Sv mice were sex-specific, extending lifespan and reducing spontaneous tumour incidence in females but reducing lifespan and having no effect on tumour incidence in males( Reference Anisimov, Piskunova and Popovich 113 ). Similar to DR, the age at which metformin treatment is started appears important to any lifespan effects. In female outbred Swiss-derived mice, lifespan extension was seen when metformin treatment was initiated at age 3 months, but not when initiated at age 15 months( Reference Anisimov, Berstein and Popovich 114 ). In this same study, metformin delayed age-related irregularities in oestrous cycle, although no effect on serum glucose, insulin and total cholesterol or TAG levels was reported( Reference Anisimov, Berstein and Popovich 114 ). In mice, 8 weeks metformin treatment recapitulate the transcriptional effects of long-term DR in liver more rapidly than 8 weeks DR( Reference Dhahbi, Mote and Fahy 83 ). Metformin also extends lifespan in C. elegans ( Reference Onken and Driscoll 115 , Reference Cabreiro, Au and Leung 116 ); with the most recent of these studies demonstrating that these effects are mediated through alterations in folate and methionine metabolism in the worm's Escherichia coli food source. This suggests that the efficacy of metformin on lifespan may be dependent on exactly how it impacts on the gut microbiota( Reference Cabreiro, Au and Leung 116 ). Metformin also promotes neurogenesis and improves spatial memory in adult mice( Reference Wang, Gallagher and DeVito 117 ), reverse fatty liver disease in ob/ob mice( Reference Lin, Yang and Chuckaree 118 ), slows sarcopaenia in elderly men( Reference Lee, Boyko and Barrett-Connor 119 ) and is associated with a reduced risk of cancer in type 2 diabetics( Reference Lin, Kachingwe and Lin 120 ).
However, the effects of metformin on longevity are not universal. In Fischer-344 rats, despite metformin treatment reducing body mass and food intake relative to controls, no effect on lifespan was reported( Reference Smith, Elam and Mattison 121 ). Similarly, while metformin in Drosophila both reduced lipid stores and activated AMPK, no effect on lifespan was reported in either male or female flies( Reference Slack, Foley and Partridge 122 ). Metformin treatment also did not rescue the lifespan of the SOD1 (G93A) mouse model of amyotrophic lateral sclerosis( Reference Kaneb, Sharp and Rahmani-Kondori 123 ), although did increase lifespan of male (but not female) Huntington's disease mice( Reference Ma, Buescher and Oatis 124 ). Finally, in contrast to rapamycin, resveratrol and several other compounds, metformin does not appear to have any effect on the generation of pluriponent stem cells in mice( Reference Chen, Shen and Yu 125 ).
Resveratrol
Perhaps no compound has created greater debate on its effects on lifespan and health, on its precise mode of action and on its potential as a DR mimetic as the polyphenol resveratrol has( Reference Fontana, Partridge and Longo 126 – Reference Baur, Ungvari and Minor 128 ). Resveratrol has been shown to increased lifespan in yeast, C. elegans and Drosophila apparently through the activation of NAD-dependent histone deacetylases called sirtuins( Reference Howitz, Bitterman and Cohen 129 , Reference Wood, Rogina and Lavu 130 ), with SIRT1 activation reported to underlie the effects of DR in mammals( Reference Wood, Rogina and Lavu 130 ). In male C57BL/6 mice fed a high-fat diet (HFD), combined with resveratrol supplementation from age 12 months showed increased lifespan relative to HFD-only fed mice( Reference Baur, Pearson and Price 131 ). In terms of healthspan, resveratrol has been shown to increase insulin sensitivity, improve motor function, induce mitochondrial biogenesis, increase running time and prevent development of fatty liver in mice( Reference Baur, Pearson and Price 131 – Reference Lagouge, Argmann and Gerhart-Hines 133 ). In addition, hepatic transcriptional profiles in the HFD animals supplemented with resveratrol were shifted back towards those of the chow-fed controls( Reference Baur, Pearson and Price 131 ). Resveratrol treatment has also been reported to reduce neurodegeneration in Alzheimer's mouse models( Reference Kim, Nguyen and Dobbin 134 ), and extend lifespan in both the annual fish Notobranchi guenther ( Reference Yu and Li 135 ) and in honey bees Apis mellifera ( Reference Rascon, Hubbard and Sinclair 136 ). Excitingly, even supplementation over 30 d with resveratrol in obese human subjects induced significant DR-like effects on metabolism( Reference Knop, Konings and Timmers 137 , Reference Timmers, Konings and Bilet 138 ). However, in contrast, other studies have reported no effects on lifespan following resveratrol treatment in C. elegans or Drosophila ( Reference Bass, Weinkove and Houthoofd 139 ) or any effect following dSir2 overexpression( Reference Burnett, Valentini and Cabreiro 140 ). In addition, resveratrol supplementation from age 12 months in mice maintained on normal chow did not increase lifespan, although the incidence of several age-related pathologies, including aortic stiffening and cataracts, was slowed and/or reduced( Reference Pearson, Baur and Lewis 132 , Reference Strong, Miller and Astle 141 , Reference Miller, Harrison and Astle 142 ). In contrast to the effects of DR( Reference Lee, Duan and Long 143 ), resveratrol decreased the proliferative capacity and survival of neural progenitor cells in the hippocampal dentate gyrus of mice, and was associated with diminished cognitive function( Reference Park, Kong and Yu 144 ).
Alternate-day-fasting and the manipulation of dietary macro/micronutrients
It should be noted that alternative protocols perhaps more ‘palatable’ to human subjects can also induce wide-spectrum benefits to lifespan and health in rodents. For example, alternate-day-fasting, a protocol whereby animals have AL access to food for 24 h and then no access to food for 24 h, reduces serum glucose, serum insulin and protects neurones against excitotoxic stress in mice, despite average food intake and body mass mirroring those of AL controls( Reference Anson, Guo and de Cabo 145 ). Short periods of alternate-day-fasting (4 months) initiated in middle-age in mice significantly reduced lymphoma incidence and mitochondrial reactive oxygen species( Reference Descamps, Riondel and Ducros 146 ). Alternate-day-fasting also increased lifespan in three distinct mouse strains when initiated at age 1·5 months, although complex age and strain interactions were observed when initiated later in life( Reference Goodrick, Ingram and Reynolds 147 ). Interestingly mice given only 8 h of access to a HFD daily ate equivalent calories to animals with free access to the same HFD but were protected against a range of pathologies including hepatic steatosis, hyperinsulinaemia and obesity( Reference Hatori, Vollmers and Zarrinpar 148 ). In addition, short-term DR (days/weeks) causes a transcriptional shift mirroring long-term caloric restriction in a range of tissues in mice( Reference Dhahbi, Kim and Mote 61 , Reference Selman, Kerrison and Cooray 149 ), and can have wide-ranging benefits to aspects of physiology( Reference Hempenstall, Picchio and Mitchell 51 , Reference Mulligan, Stewart and Saupe 150 ). In adulthood, dietary shifts from DR to AL (or AL to DR) in Drosophila ( Reference Mair, Goymer and Pletcher 151 ) and rats( Reference Yu, Masoro and McMahan 152 ) and shifts from AL to DR in mice( Reference Dhahbi, Kim and Mote 61 ) rapidly alters the mortality risk to that the dietary group the animals are switched to. A significant memory effect to both early-life and adult-onset DR has been also been reported in mice, particularly on glucose tolerance, even 1 year following a dietary switch back to AL feeding( Reference Cameron, Miwa and Walker 153 , Reference Selman and Hempenstall 154 ). Manipulations in specific dietary micronutrients, specifically amino acids, can also extend lifespan and improve aspects of health in animals including rodents( Reference Miller, Buehner and Chang 3 , Reference Richie, Leutzinger and Parthasarathy 155 , Reference Orentreich, Matias and DeFelice 156 ), and excitingly research recently uncoupled the effects of DR on lifespan and fecundity in Drosophila through manipulation of single amino acids( Reference Grandison, Piper and Partridge 2 ).
Conclusion
It is highly unlikely that the majority of human beings could actually commit, or want to commit, to years of reduced food intake given the profound levels of restraint required. However, for the vast majority of us it may be that all is not lost given that several realistic DR mimetics have been identified. These may ultimately lead to the generation of compounds that can provide some/all of the beneficial effects of DR without any need to modulate diet. However, it is also clear that a note of caution should be sounded before there is a scramble to prescribe rapamycin, metformin or resveratrol whole-sale to human subjects. It is clear that we need to firstly understand the mechanisms through which these potential DR mimetics work, and get a better feel for why the reported species-, strain- and gender-specific effects exist both following DR and following supplementation with these compounds. This knowledge should help in the design of safer analogues( Reference Lamming, Ye and Sabatini 90 ), or compounds which act on downstream targets, e.g. PF-4708671 that inhibits S6 kinase( Reference Pearce, Alton and Richter 157 ). In addition, interventions that limit food intake periodically or manipulate dietary components without affecting energy intake also look promising. However, these objectives are not straightforward, particularly given that while a century has passed since the first report came out that DR extends lifespan( Reference Osborne, Mendel and Ferry 6 ), we have still not identified the primary mechanism underlying DR. In particular, using comparative approaches to understand how DR works, using resources such as the ILXISS recombinant mice and non-model organisms, should help dissect out the mechanisms underlying DR. It is clear that this field of research is moving apace and so we should retain optimism that safer DR mimetics, which will extend vitality in old age, can be developed at some point in the future.
Acknowledgement
The BBSRC had no role in the design, analysis or writing of this article.
Financial support
This work was supported by the BBSRC [grant no. BB/H012850/2].
Conflicts of interest
None.