Overview and basic biology of vitamin D: sources, metabolism and function
Diet
There are two main forms of vitamin D, namely vitamin D2 (ergocalciferol) and vitamin D3 (cholecalciferol), both of which are found in the diet in predominantly plant-based and animal-based foods, respectively. While there are few foods which are a natural-rich source of vitamin D, good sources include oily fish and egg yolks (vitamin D3) and wild mushrooms (vitamin D2)(Reference Holick1). Foods can also be fortified, on a voluntary or mandatory basis, with vitamin D2 or D3, although fortification policies vary quite considerably between countries, with minimal voluntary fortification found in the UK and across much of Europe (with the exception of Finland; for review see Kiely and Black(Reference Kiely and Black2)). Consequently, dietary vitamin D intakes among adolescents are typically low (<3 µg/d). In the UK National Diet and Nutrition Survey (NDNS) rolling programme (2008/09–2011/12), mean vitamin D intakes from food sources alone were 1·9 and 2·4 µg/d in females and males aged 11–18 years, respectively(Reference Bates, Lennox and Prentice3). Main food group contributors were meat and meat products (35 %), fat spreads (20 %) and cereal/cereal products (17 %), with eggs and oily fish, both good sources of vitamin D, contributing only 9 % each, emphasising the low consumption of oily fish among adolescents(Reference Bates, Lennox and Prentice3). While supplement use can be an effective means of increasing dietary vitamin D intakes, uptake tends to be greater among infants, young children and elderly adults and low among adolescents. Indeed, in the UK NDNS, only 8 % of adolescents aged 11–18 years reported taking any type of supplement (compared with 16, 22 and 41 % of 4–10, 19–64 and 65+ year olds, respectively) and mean vitamin D intakes increased only marginally to 2·1 and 2·6 µg/d in females and males, respectively, when supplements were included(Reference Bates, Lennox and Prentice3).
Endogenous synthesis
Vitamin D is unique in that the majority of this nutrient (80–90 %) is synthesised within the human body following skin exposure to sunlight, hence vitamin D's recognition as the ‘sunshine nutrient’. Ultraviolet B (UVB) radiation (wavelength 290–315 nm) mediates the conversion of 7-dehydrocholesterol, a cholesterol precursor contained within the skin, to vitamin D3. This synthesised vitamin D3, along with dietary vitamin D2/D3, then undergoes a two-step hydroxylation process: first in the liver to form the biologically inactive 25-hydroxyvitamin D (25(OH)D; the main circulating and storage form of vitamin D and that measured to assess vitamin D status), and secondly in the kidneys to the active 1,25-dihydroxyvitamin D (1,25(OH)2D) when required by the body(Reference Holick1). The amount of vitamin D3 endogenously produced is a function of the amount of UVB radiation reaching the skin, therefore being affected by a number of environmental and individual factors, including season and latitude, skin pigmentation, concealing clothing, use of sunscreen, time spent outdoors and other individual factors such as obesity and ageing(Reference Webb4). Season and latitude dramatically affect vitamin D3 synthesis, and at high latitudes >40°N during the winter time (October–March), there are marked decreases in endogenous vitamin D3 production, giving rise to striking seasonal variations in vitamin D status throughout the year(Reference Bates, Lennox and Prentice3, Reference Darling, Hart and Macdonald5, Reference Klingberg, Oleröd and Konar6). This is demonstrated in the NDNS data of sex-combined adolescents aged 11–18 years, whose mean plasma 25(OH)D concentrations ranged from 52·3 nmol/l when blood samples were collected during the months of July–September, down to 31·5 nmol/l when sampled during the winter months of January–March(Reference Bates, Lennox and Prentice3).
Physiological function
The primary and well-recognised function of the biologically active metabolite 1,25(OH)2D is in the maintenance of calcium and phosphorus homeostasis via endocrine mechanisms targeting the intestine, kidneys and bone(Reference Holick1, Reference DeLuca7). This is essential for skeletal health throughout the life cycle, from bone accretion and growth in infancy, childhood and adolescence, through to maintenance of healthy bones and prevention of bone loss in later adulthood. Vitamin D, along with calcium, is important during the adolescent years when the most rapid bone accrual occurs(Reference Weaver, Gordon and Janz8). Approximately 80–90 % of peak bone mass is achieved by late adolescence and maximising this may help reduce age-related bone loss in later life(Reference Bailey, McKay and Mirwald9, Reference Henry, Fatayerji and Eastell10).
In recent years however, more attention has been paid to the paracrine/autocrine functions of vitamin D in the facilitation of gene expression. It was previously believed that the kidneys were the only site of 1,25(OH)2D synthesis, although it is now recognised that many extra renal tissues (e.g. colon, prostate, breast and immune cells) have the ability to locally convert 25(OH)D to 1,25(OH)2D due to the presence of the vitamin D receptor and 1 α-hydroxylase enzymes(Reference DeLuca7, Reference Norman11). Epidemiological studies have therefore indicated a broader role of vitamin D in common cancers, CVD and respiratory diseases(Reference Bouillon, Schoor and Gielen12–Reference Martineau, Jolliffe and Hooper14). Although vitamin D is emerging as a promising nutrient in many extra skeletal health outcomes, it must be borne in mind that the evidence is largely observational and thus further data from robust randomised controlled trials (RCT) is required to help clarify the causal role of vitamin D and the underlying mechanisms.
Vitamin D deficiency: definitions, prevalence and consequences
Defining vitamin D deficiency and adequacy
Currently there is no international consensus on the optimal circulating 25(OH)D concentrations for health, with debate surrounding the cut-off thresholds to be applied to define vitamin D deficiency and adequacy. Table 1 summarises the current cut-off thresholds proposed by various international authoritative bodies and agencies.
Table 1. Circulating 25-hydroxyvitamin D deficiency and adequacy cut-off thresholds currently proposed by various international agencies

ESPGHAN, European Society for Paediatric Gastroenterology, Hepatology and Nutrition.
There is generally good agreement that populations should not have circulating 25(OH)D concentrations below 25–30 nmol/l based on an increased risk of rickets and impaired bone growth in adolescents. At present, the Institute of Medicine (IOM), European Food Safety Authority (EFSA), European Society for Paediatric Gastroenterology, Hepatology and Nutrition and the American Academy of Pediatrics, all suggest that a serum 25(OH)D concentration >50 nmol/l is adequate based on ensuring optimal bone health(15–Reference Wagner and Greer18), while others have proposed much greater 25(OH)D sufficiency thresholds. The Society for Adolescent Health and Medicine and the Endocrine Society, for example, consider sufficiency at 25(OH)D concentrations >75 nmol/l and that concentrations <50 nmol/l are indicative of deficiency(19, Reference Holick, Binkley and Bischoff-Ferrari20). It is worth noting however that the Endocrine Society guidelines target patient care of those at risk of vitamin D deficiency (e.g. obese patients, patients with malabsorption syndromes or those on medication that affects vitamin D metabolism), in contrast to the IOM, EFSA and the UK Scientific Advisory Committee on Nutrition (SACN), who propose recommendations for the general healthy population(15, 16, 21). Regardless it may be premature to recommend circulating 25(OH)D concentrations >75 nmol/l due to the lack of evidence from RCT to date supporting higher serum 25(OH)D concentrations and health outcomes beyond musculoskeletal health.
Prevalence of vitamin D deficiency among adolescent populations
Adolescents are a population group with a recognised risk of low vitamin D status, and several nationally representative and individual studies have highlighted the global nature of vitamin D deficiency and inadequacy among adolescent populations(Reference Smith, Lanham-New and Hart22). Estimates of vitamin D deficiency (defined across studies as 25(OH)D <22·5–27·5 nmol/l) have ranged from 4–17 % in the USA and Canada(Reference Ganji, Zhang and Tangpricha23–Reference Whiting, Langlois and Vatanparast26), 15–40 % in Europe(Reference Bates, Lennox and Prentice3, Reference González-Gross, Valtueña and Breidenassel27–Reference Öberg, Jorde and Almås31), 13–33 % in Asia (Korea, India and China)(Reference Kim, Oh and Namgung32–Reference Foo, Zhang and Zhu34) and up to 81 % in Saudi Arabia(Reference Siddiqui and Kamfar35). If a cut-off of 50 nmol/l is applied, these estimates increase to 19–91 % of the population as having inadequate vitamin D concentrations(Reference Smith, Lanham-New and Hart22). Data from the UK NDNS rolling programme (2008/09–2011/12) demonstrated that 22 % of adolescents aged 11–18 years had year-round plasma 25(OH)D concentrations <25 nmol/l and this increased to 40 % during the winter months (January–March)(Reference Bates, Lennox and Prentice3).
Caution must be taken when comparing data from different studies due to variations in participant characteristics (e.g. age, ethnicity, cultural dress), latitude and season of sampling and in the analytical techniques and assays used to measure 25(OH)D (for review of the impact of different analytical techniques on 25(OH)D results, see Fraser and Milan(Reference Fraser and Milan36)). A recent study, based on standardised serum 25(OH)D data from European cohorts and national surveys (n 55 844), found adolescents aged 15–18 years to have the highest risk of vitamin D deficiency (25(OH)D <30 nmol/l) in the range of 12–40 %, compared with 4–7 % in younger children (1–14 years), 9–24 % in adults (19–60 years) and 1–8 % in older adults (>61 years)(Reference Cashman, Dowling and Škrabáková37). The underlying causes of this apparent increased risk and higher prevalence of vitamin D deficiency in adolescents are unclear, although negative associations between pubertal status and circulating 25(OH)D concentrations have been reported(Reference El-Hajj Fuleihan, Nabulsi and Choucair38–Reference Abrams, Hicks and Hawthorne40), which may be due to behavioural (e.g. reduced outdoor time, sedentary lifestyles)(Reference Absoud, Cummins and Lim41) and/or biological mechanisms(Reference Aksnes and Aarskog42). In order to meet the increased calcium demands for skeletal growth during adolescence, the metabolism of 25(OH)D to the active 1,25(OH)2D is increased(Reference Aksnes and Aarskog42) and a concomitant decrease in circulating 25(OH)D has been reported(Reference Aksnes and Aarskog42, Reference Lehtonen-Veromaa, Möttönen and Irjala43), although not consistently(Reference Krabbe, Christiansen and Hummer44).
Consequences of vitamin D deficiency and inadequacy during adolescence
This susceptibility to low vitamin D status in adolescent populations is concerning given the important role of vitamin D in promoting normal bone mineralisation and attainment of peak bone mass during periods of rapid growth and development. It is well recognised that prolonged and severe vitamin D deficiency leads to an increased risk of rickets and altered bone development in children and adolescents(Reference Pettifor45), although the effects of mild vitamin D inadequacy (25(OH)D between 30 and 50 nmol/l), which has been widely reported in adolescent populations worldwide(Reference Wahl, Cooper and Ebeling46), remains less clear. Observational studies in adolescent females have suggested adverse effects of circulating 25(OH)D concentrations <40–50 nmol/l on bone mass at various skeletal sites(Reference Outila, Kärkkäinen and Lamberg-Allardt29, Reference Cheng, Tylavsky and Kröger30, Reference Cashman, Hill and Cotter47, Reference Foo, Zhang and Zhu48), although the evidence is inconsistent with others reporting no association between vitamin D status and bone mass in adolescents(Reference Kristinsson, Valdimarsson and Sigurdsson49, Reference Marwaha, Tandon and Reddy50). A 3-year prospective study in Finnish females aged 9–15 years found a 4 % difference in lumbar spine bone mineral density accrual between those with serum 25(OH)D concentrations >37·5 nmol/l and those with concentrations <20 nmol/l at baseline(Reference Lehtonen-Veromaa, Möttönen and Nuotio51). Importantly, there is limited data available in male adolescents, a research gap recognised in the National Osteoporosis Foundation's 2016 position statement on peak bone mass development(Reference Weaver, Gordon and Janz8). Intervention studies, predominantly in white females, have failed to consistently find a beneficial effect of vitamin D supplementation on bone health indices in adolescents and a 2010 Cochrane systematic review concluded that supplementation may only benefit those with inadequate circulating 25(OH)D concentrations below 35 nmol/l(Reference Winzenberg, Powell and Shaw52). There is currently no consensus on the 25(OH)D concentration that is optimal for the acquisition of bone mass in adolescents or the outcome measures that should be targeted.
As previously alluded to, there is a growing body of evidence that vitamin D may be associated with other non-musculoskeletal, chronic diseases, such as CVD, cancer, diabetes and autoimmune diseases. However, much of the evidence base for this has been derived from epidemiologic studies of adults and much less is known of the effect of low vitamin D status during adolescence on these health outcomes in the short and long terms(Reference Holick53). Cross-sectional studies in children and adolescents aged 2–19 years have found circulating 25(OH)D concentrations to be inversely associated with total and LDL-cholesterol concentrations(Reference Petersen, Dalskov and Sørensen54, Reference Cabral, Araújo and Teixeira55), glucose(Reference Reis, von Mühlen and Miller56, Reference Johnson, Nader and Weaver57) and systolic blood pressure(Reference Petersen, Dalskov and Sørensen54, Reference Reis, von Mühlen and Miller56, Reference Ganji, Zhang and Shaikh58, Reference Williams, Fraser and Lawlor59) and positively correlated with HDL-cholesterol(Reference Ganji, Zhang and Tangpricha23, Reference Johnson, Nader and Weaver57, Reference Williams, Fraser and Lawlor59), although not consistently(Reference Nam, Kim and Cho60, Reference Black, Burrows and Lucas61). Furthermore, vitamin D status below 50 nmol/l has been associated with an increased prevalence of metabolic syndrome in adolescents aged 12–19 years in the USA(Reference Reis, von Mühlen and Miller56, Reference Ganji, Zhang and Shaikh58); however, no association was found in Portuguese adolescents aged 13 years(Reference Cabral, Araújo and Teixeira55). A 2013 systematic review concluded that there was no consistent association between 25(OH)D and lipid and glucose concentrations in adolescents and that systolic blood pressure was inversely associated with 25(OH)D in cross-sectional studies, but no association was found in prospective cohort studies(Reference Dolinsky, Armstrong and Mangarelli62).
Current vitamin D recommendations
From a public health perspective, dietary vitamin D recommendations are of great importance in helping to prevent vitamin D deficiency, particularly during the winter months when UVB exposure is inadequate for cutaneous synthesis. Many countries and authoritative bodies worldwide have proposed vitamin D intake recommendations and some have recently been re-evaluated and revised (Table 2). Due to difficulties in establishing the contribution of UVB exposure to vitamin D status, such recommendations are often set assuming minimal sun exposure and are the average daily intakes needed to meet the requirements of the majority of the population (i.e. the vitamin D intake needed to maintain serum 25(OH)D concentrations above the specified threshold in 97·5 % of the population). Furthermore, these recommendations are based upon intakes that will ensure adequate vitamin D status to protect against poor musculoskeletal health and do not, at this stage, consider the prevention or reduction in risk of non-musculoskeletal health outcomes. Additionally, recommendations can vary between different life stage groups, with vitamin D supplementation specifically recommended for infants, pregnant and lactating women and the elderly, and are often determined based on the evidence from white Caucasian populations. As demonstrated in Table 2, at present there is a lack of consensus on the intakes required to maintain circulating 25(OH)D concentrations above differing levels of defined adequacy.
Table 2. Dietary reference values for vitamin D (μg/d) by life stage as proposed by various international agencies to maintain adequate circulating 25-hydoxyvitamin D (25(OH)D) concentrations
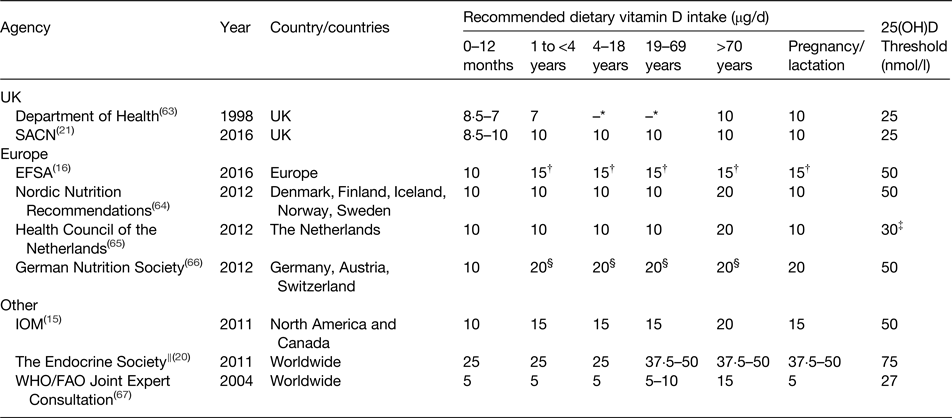
SACN, Scientific Advisory Committee on Nutrition; EFSA, European Food Safety Authority; IOM, Institute of Medicine.
* For those aged 4–64 years, it was assumed that sun exposure during the summer months would ensure an adequate vitamin D status year-round, so no intake was set.
† Requirements set under conditions of minimal cutaneous synthesis and in the presence of cutaneous vitamin D3 synthesis the requirement for dietary vitamin D is lower or may even be zero.
‡ Target concentration of 50 nmol/l for those aged >70 years.
§ Estimated value of adequate vitamin D intake without cutaneous synthesis.
‖ Recommendations for populations at risk of vitamin D deficiency.
Development and re-evaluation of the UK recommendations
In 1991, the UK Department of Health published dietary reference values for most nutrients and energy(68). At this time, it was assumed that free-living individuals aged between 4 and 64 years of age would synthesise sufficient vitamin D from summer sun exposure to ensure adequate vitamin D throughout the winter months to avoid deficiency (25(OH)D <25 nmol/l). Thereby no reference nutrient intake was set for these age groups, although intake recommendations were determined for population sub-groups perceived to be at risk of vitamin D deficiency, namely infants, those aged ≥65 years and pregnant and lactating women (Table 2). Subsequent reviews in 1998 and 2007 by the Department of Health and SACN, respectively, concluded that these recommendations should remain unchanged while further evidence was generated from RCT(63, 69). In 2010, SACN agreed to reassess the evidence relating to vitamin D and health on the basis of new data that had become available since the previous review.
The revised dietary intake recommendations for vitamin D were released by SACN in 2016 and a daily intake of 10 µg was set for those aged ≥4 years to ensure a serum 25(OH)D concentration >25 nmol/l, which was deemed protective against adverse musculoskeletal health outcomes(21). This year-round recommendation also includes those of ethnic minority groups and with limited sun exposure (e.g. institutionalised individuals). A precautionary safe intake of 8·5–10 µg/d, rather than a reference nutrient intake, was set for infants 0 to <4 years of age due to insufficient evidence and uncertainties with specifying an intake recommendation for this age group.
Other notable recommendations
The 2011 IOM dietary reference intakes was the first landmark publication of vitamin D (and calcium) requirements, based on a risk assessment framework and provided a comprehensive review of vitamin D requirements for health(15). The risk assessment framework, which has previously been reviewed in detail(Reference Cashman and Kiely70, Reference Cashman and Kiely71), has since been adopted in more recent re-evaluations of vitamin D requirements by EFSA, SACN and the Nordic Nutrition Recommendations(16, 21, 64). Briefly, the risk assessment framework involves undertaking independent systematic evidence-based reviews, which are then used by the committee to identify, describe and rate potential indicators (e.g. rickets, osteomalacia, calcium absorption and fracture risk) in order to derive population targets for 25(OH)D status. The associated dietary vitamin D intake requirements were then established by the IOM via a simulated dose–response meta-regression exercise using group mean/median 25(OH)D response data from selected RCT (winter-based at latitudes >49°N)(15). Based upon this method, the IOM committee proposed a RDA of 15 µg/d for those aged 1–70 years (20 µg/d for those >70 years), which corresponds to a serum 25(OH)D concentration of 50 nmol/l that meets the needs of 97·5 % of the population. Additionally, an estimated average requirement (EAR) of 10 µg/d for those aged ≥1 year was set to maintain serum 25(OH)D of 40 nmol/l in 50 % of the population(15).
Interestingly, using the same simulated dose–response meta-regression method, the Nordic Nutrition Recommendations set a recommended intake (RDA equivalent) of 10 µg/d to maintain serum 25(OH)D >50 nmol/l, using data from winter-based RCT conducted at latitudes covering the Nordic region (49·5–60°N)(64).
Of note are also the recent EFSA vitamin D dietary reference values (15 µg/d to maintain serum 25(OH)D >50 nmol/l), which defined an adequate intake instead of an average requirement, based on the committee's assertion that there was insufficient evidence to allow for an average requirement to be established for all population groups(16).
In contrast to the meta-regression approach adopted by the IOM, EFSA and the Nordic Nutrition Recommendations as described earlier, SACN generated its vitamin D intake estimates by modelling individual participant-level data and it has been suggested that this method may lead to improved dietary requirement guidelines(Reference Vale, Rydzewska and Rovers72, Reference Cashman, Ritz and Kiely73). This may also be an underlying reason for the variability in the current recommendations and is discussed in more detail later.
Meta-regression v. individual participant-level modelling in determining vitamin D intake requirements
The use of meta-regression and individual participant-level modelling and the impacts on dietary vitamin D intake estimates has been described previously in detail(Reference Cashman, Fitzgerald and Kiely74, Reference Cashman75) and so will be reviewed briefly here. The meta-regression approach used by the IOM, and more recently EFSA, in establishing vitamin D requirements used group mean/median data from intervention arms of selected RCT, together with estimates of vitamin D intakes (from diet and supplements) in a simulated dose–response relationship(15, 16). While this avoids over-reliance on data from any particular RCT, the disadvantage is that data are combined from different RCT that use a variety of analytical methods to measure 25(OH)D concentrations(Reference Fraser and Milan36). The use of group mean/median data and the resulting regression line and 95 % CI in the meta-regression model generates average responses, and as such the intake estimates of 10 and 15 µg/d might only be expected to offer protection for 50 % of the population (EAR-type estimate) instead of the intended 97·5 % (RDA-type estimate). Furthermore, the meta-regression approach does not take into consideration the inter-individual variability in 25(OH)D response to increasing vitamin D intakes. This can be overcome with the use of individual participant-level data and 95 % prediction intervals (Fig. 1), as applied by SACN(21). Individual data from three winter-based RCT in female adolescents aged 11–12 years(Reference Cashman, FitzGerald and Viljakainen76) and adults aged 20–40 and ≥64 years(Reference Cashman, Hill and Lucey77, Reference Cashman, Wallace and Horigan78) was modelled by SACN in order to derive vitamin D intake estimates for the UK population. With this method, it is possible to estimate with more confidence the distribution of intakes required to achieve specific serum 25(OH)D concentrations.

Fig. 1. Relationship between serum 25-hydroxyvitamin D (25(OH)D) concentration and total vitamin D intake using data from randomised controlled trials. The central thick solid line is the regression line and the two curved lines represent the 95 % CI around the mean. The outer two dashed lines represent the 95 % prediction intervals. IU, international units (to convert IU to μg/d divide by 40). Reprinted from Journal of Steroid Biochemistry and Molecular Biology, 148, Cashman KD, Vitamin D: dietary requirements and food fortification as a means of helping achieve adequate vitamin D status, 19–26, Copyright (2015), with permission from Elsevier.
Evidence-based dietary vitamin D requirements in adolescence: use of individual participant-level data to fill knowledge gaps
While vitamin D intake recommendations have been set for the adolescent age group (Table 2), the regulatory authorities tasked with establishing these recommendations have highlighted the significant knowledge gaps in relation to winter-based dose–response RCT specifically designed to estimate dietary vitamin D intakes required to maintain 25(OH)D above certain cut-off thresholds in younger populations. Consequently, intake recommendations for adolescents are often extrapolated from adult data in the absence of adolescent-specific data. Of the three RCT in children and adolescents included in the IOM dose–response model, a 1 month long study was conducted in twenty participants (6–14 years)(Reference Schou, Heuck and Wolthers79) and another was carried out in 1988 in sixty participants (8–10 years) using doses of 0 and 10 µg/d(Reference Ala-Houhala, Koskinen and Koskinen80). Other under-researched population sub-groups identified with a lack of dose–response experimental data also included younger children, pregnant women and ethnic minority populations. Winter-based dose–response RCT, designed and implemented within the Food-based solutions for optimal vitamin D nutrition through the life cycle (ODIN) project aimed to fill these knowledge gaps by modelling individual participant-level data in order to estimate dietary requirements for vitamin D in these population sub-groups(Reference Kiely and Cashman81).
In the ODIN adolescent study, 110 healthy white males and females aged 14–18 years were randomly allocated to receive 0, 10 or 20 µg vitamin D3 daily for 20 weeks throughout the winter (October–March)(Reference Smith, Tripkovic and Damsgaard82). Via mathematical modelling of individual participant-level 25(OH)D and total vitamin D intake data, the vitamin D intakes required to maintain serum 25(OH)D concentrations above 25, 30, 40 and 50 nmol/l in 50, 90, 95 and 97·5 % of the adolescents were estimated. Of particular note, the vitamin D intakes needed to avoid deficiency and maintain serum 25(OH)D concentrations >25 and >30 nmol/l in 97·5 % of the population were 10·1 and 13·1 µg/d, respectively(Reference Smith, Tripkovic and Damsgaard82). The 10·1 µg/d estimate supports the SACN recommendation for the UK population aged ≥4 years. In order to maintain serum 25(OH)D >40 nmol/l in 50 % of the population, corresponding to the EAR-type intake, 6·6 µg/d was estimated for adolescents. While lower than the 10 µg/d recommendation of the IOM, this is close agreement with the 6·3 µg/d estimate in Danish and Finnish females aged 11–12 years in a similarly designed RCT(Reference Cashman, FitzGerald and Viljakainen76). However, greater discrepancies arise when considering the RDA-type intake (to maintain serum 25(OH)D >50 nmol/l in 97·5 % of the population). The intake estimate of about 30 µg/d in adolescents derived from the ODIN study is considerably higher than the IOM recommendation of 15 µg/d. The large inter-individual variability in 25(OH)D response to vitamin D intakes and the inability of the meta-regression using aggregate data to consider this, explains, in part, the wide variation in these estimates at the 97·5th percentile(Reference Cashman, Ritz and Kiely73).
It is important to note that the adolescent trial aforementioned and many of those included by the IOM, SACN and EFSA were conducted in healthy white populations. A winter-based RCT in 8–14 year old black African American and white children and adolescents estimated that vitamin D intakes of 28 and 52 µg/d would maintain serum 25(OH)D >30 and >50 nmol/l, respectively, in 97·5 % of the population(Reference Rajakumar, Moore and Yabes83). Unfortunately intake estimates were not determined for the ethnic groups separately, limiting the opportunity to compare intake estimates. In a recent food-based dose–response RCT in Swedish children aged 5–7 years, it was estimated, using 95 % prediction intervals, that vitamin D intakes of 6 and 20 µg/d would maintain 25(OH)D >30 and >50 nmol/l, respectively, in fair-skinned children, while the corresponding intakes in dark-skinned children were 14 and 28 µg/d, respectively(Reference Öhlund, Lind and Hernell84). These data provide early indications that greater intakes may be required by ethnic minority populations; however, this should be confirmed in further winter-based vitamin D dose–response RCT(Reference Smith and Hart85).
Achieving vitamin D intakes: supplements v. food fortification
Current recommendations, along with the new data presented here, propose that vitamin D intakes of between 10 and about 30 µg/d are required by adolescents in order to avoid vitamin D deficiency and ensure adequacy. Population-based dietary intake surveys in the UK and beyond have highlighted significant gaps between typical vitamin D intakes in adolescents and these recommendations, especially in countries with limited food fortification, which includes the UK. Current intakes in UK adolescents aged 11–18 years participating in the NDNS were about 2 µg/d(Reference Bates, Lennox and Prentice3). Strategies therefore need to be implemented in order to help the UK population achieve higher dietary vitamin D intakes and this becomes increasingly important during times of insufficient UVB exposure for cutaneous synthesis. While vitamin D supplements can be useful in increasing vitamin D intakes and consequently vitamin D status, relying on supplements may not be a viable public health strategy to increase intakes at the population level. Supplement use is not widespread and will only be effective in those that take them, which varies by age, sex and by individuals’ health motivation and purchasing power. As previously mentioned, supplement uptake is typically low among adolescents compared with younger and older age groups, and so adolescents are far less likely to acquire the same level of benefit as other population groups do from supplements. Therefore, sustainable public health strategies to ensure vitamin D intakes need to be designed and implemented to continually meet the needs of the majority, irrespective of age, sex and other individual factors.
Food fortification presents an opportunity to increase vitamin D intakes at the population level. Using data from the 2003–2006 National Health and Nutrition Examination Survey in the USA, fortification has been shown to considerably improve dietary vitamin D intakes above that obtained from the basal diet(Reference Fulgoni, Keast and Bailey86). Intakes of 1·7 µg/d were reported in children aged 2–18 years and adolescents from naturally occurring food sources, with 100 % having intakes below the IOM EAR of 10 µg/d. Intakes increased to 6·1 µg/d when fortified foods were included, with 86·8 % having intakes below the EAR. Commonly fortified foods in the USA include milk, breakfast cereals, yoghurts, cheeses, juices and spreads. When supplement use was also considered, intakes further increased to 8·3 µg/d, with 73·2 % of intakes remaining below 10 µg/d. However, dietary diversity needs to be given important consideration when developing and initiating fortification policies. While fortification of milk and other staple commodities (e.g. margarine/fat spreads, other dairy products and breakfast cereals) will be important for increasing vitamin D in the food supply, this will not be an effective strategy in non- or infrequent consumers. For example, routine fortification of milk with vitamin D in Finland had little impact on the vitamin D intakes of female adolescents (12–18 years)(Reference Lehtonen-Veromaa, Möttönen and Leino87). Intakes increased from 4·0 µg/d prior to the introduction of the fortification policy to 5·4 µg/d following fortification introduction, with no change in serum 25(OH)D concentrations (48·3 and 48·1 nmol/l, respectively)(Reference Lehtonen-Veromaa, Möttönen and Leino87). Additionally the prevalence of serum 25(OH)D concentrations <50 nmol/l in these adolescent females was 60·6 and 65·5 % prior to and following fortification, respectively. Conversely fortification of milk and fat spreads in Finland was found to have a positive impact on the dietary vitamin D intakes of adults and young children(Reference Laaksi, Ruohola and Ylikomi88–Reference Soininen, Eloranta and Lindi91). This may be due to low and/or infrequent consumption of milk and other dairy products among adolescent females. These data from Finland demonstrate the importance of giving consideration to food consumption patterns in fortification policies and several widely consumed foods should be fortified to ensure widespread reach. Dietary diversity among different ethnic groups can also influence vitamin D intakes, with African American adolescent females reported to consume more vitamin D from meat and bean food sources compared with white females who consumed more from milk, which is routinely fortified in the USA(Reference Van Horn, Bausermann and Affenito92). Bio-fortification of food presents a novel opportunity to increase vitamin D in the food supply through a range of foods, alongside more traditional fortification practices. The vitamin D content of animal produce (e.g. pork, beef, chicken, eggs and fish) can be increased via the fortification of livestock feeds, where permissible. RCT conducted within the aforementioned ODIN project have initiated investigation into the efficacy and safety of vitamin D fortification and bio-fortification of a variety of foodstuffs in different population groups and we await these findings(Reference Kiely and Cashman81).
Conclusions
Vitamin D recommendations are of great importance from a public health perspective in terms of preventing vitamin D deficiency and optimising vitamin D status for health. It is crucial therefore that recommendations are evidence-based in order to establish more accurate and precise requirements for population sub-groups. Limited experimental data continue to be an issue for certain population sub-groups, particularly those of ethnic minority populations, and further research and targeted dose–response RCT should be undertaken to continue to fill these gaps in the evidence base. This will have important implications for public health policy as presented here: whilst the current recommendations of 10–15 µg/d may help avoid winter-time vitamin D deficiency in adolescents (circulating 25(OH)D concentrations <25–30 nmol/l), they remain inadequate to achieve and maintain 25(OH)D concentrations above 50 nmol/l. This upper threshold of circulating 25(OH)D concentration may be optimal for health, particularly with respect to bone accretion in adolescents, although this needs to be confirmed in RCT, along with other non-musculoskeletal health outcomes. Consideration should also be given to the model adopted for the estimation of dietary requirements for vitamin D and future refinements of these should consider the use of individual participant-level data from dose–response RCT as more data become available. Understanding optimal vitamin D concentrations, clinical outcomes and vitamin D intake requirements will help prioritise and inform public health strategies to prevent vitamin D deficiency.
Acknowledgments
T. J. S. would like to acknowledge the European Commission's Seventh Framework Programme (FP/2007–2013) for funding her PhD studentship. The authors would like to acknowledge the ODIN Work Package 4 collaborators: Professors Camilla T Damsgaard and Christian Mølgaard (University of Copenhagen, Denmark); and Professors Mairead Kiely and Kevin D Cashman (University College Cork, Ireland).
Financial Support
The work leading to this was funded by the European Commission under its Seventh Framework Programme (FP/2007–2013) under Grant Agreement 613977 for the ODIN project (Food-based solutions for Optimal vitamin D Nutrition and health through the life cycle; www.odin-vitd.eu/).
Conflicts of Interest
None.
Authorship
T. J. S. wrote the manuscript; L. T., S. A. L.-N. and K. H. H. assisted with manuscript editing; T. J. S. had primary responsibility for the final content; all authors read and approved the final manuscript.