- ARA
-
arachidonic acid
- COX
-
cyclooxygenase
- LOX
-
lipoxygenase
- PDX
-
protectin DX
DHA (22 : 6n-3) is an abundant long-chain PUFA of marine origin( Reference Ackman 1 ). In addition to being a major PUFA of the brain and retina in animals( Reference Bazan, Gordon and Rodriguez de Turco 2 ), DHA is well-known as a relevant nutrient which prevents adverse cardiovascular events( Reference Leaf and Kang 3 ). Together with its precursor EPA (20 : 5n-3), another major component of marine lipids( Reference Weaver and Holub 4 ), DHA is one of the two main n-3 long-chain PUFA believed to be responsible for protection against cardiovascular events( Reference Dyerberg and Jørgensen 5 ). However, it is worth mentioning the possible involvement in these biological events of the third most abundant component in marine lipids( Reference Joensen, Schmidt and Dethlefsen 6 ), the intermediate between EPA and DHA, docosapentaenoic acid (22 : 5n-3), and that of the n-3 family precursor α-linolenic acid (18 : 3n-3)( Reference Mozaffarian 7 ).
During the last decade, a series of oxygenated derivatives of DHA have been described with activities against inflammation, some speeding the resolution phase of inflammation, then playing an interesting role in the prevention of atherogenesis. The oxygenated metabolites have been named protectins and resolvins( Reference Ariel and Serhan 8 , Reference Schwab, Chiang and Arita 9 ). One protectin made by macrophages has been named maresin( Reference Serhan, Yang and Martinod 10 ). Lipoxygenases (LOX) are key enzymes in the generation of these derivatives, which makes the action of DHA quite specific and different from that of EPA that is active through some of its cyclooxygenase (COX) products as well. In the 1980s, DHA had already been reported as a fairly good substrate of blood platelet 12-LOX( Reference Aveldaño and Sprecher 11 ). Also, its mono-hydroxylated metabolites produced through LOX have been found to be inhibitors of the thromboxane-induced platelet aggregation as well as blood vessel constriction( Reference Croset, Sala and Folco 12 ).
This short review will consider the ex vivo effect of dietary DHA used at low dosage, and the in vitro biological effects of a double LOX product of DHA called protectin DX (PDX), in the context of the cardiovascular risk.
DHA supplementation in human subjects
A large number of trials have been conducted with long-chain n-3 PUFA with various proportions of EPA and DHA, and also docosapentaenoic acid n-3 when more or less crude fish oil or fish meat were used for supplementing the diet. It is generally assumed that those supplementations have preventive effects against cardiovascular events. However, depending on the status of volunteers (age, dietary habits, possible usage of drugs, etc.) the benefits of such supplementations may be controversial. Indeed, a recent meta-analysis that did not take into account the different situations of the people participating in the trials concluded that ‘omega-3 PUFA supplementation was not associated with a lower risk of all-cause mortality’ ( Reference Rizos, Ntzani and Bika 13 ). A post-review further indicates that ‘Subgroup analyses suggested that this could be because of a low absolute risk as a consequence of the state-of-the-art drug treatment’( Reference Kromhout 14 ).
Earlier, we considered that the dosage in EPA–DHA supplementation could be an issue as their high level of unsaturation makes them highly susceptible to peroxidation that could be detrimental to their potential benefit. Relating to blood platelets that play a crucial role in the initiation of atherothrombogenesis, we first considered that the increased oxidative stress associated with ageing( Reference Véricel, Croset and Sedivy 15 ) could negatively affect the expected benefit of the intake of long-chain n-3 PUFA. We conducted an assay with a small dosage of those (150 mg DHA+30 mg EPA esterified in TAG), daily for 6 weeks. The main results at the platelet level were a significant accumulation of DHA in membrane ethanolamine phospholipids and an increase in platelet vitamin E, the latter being of interest because platelet vitamin E is lower in this population of elderly people compared with young adults, associated with a decrease in malondialdehyde concentration( Reference Véricel, Calzada and Chapuy 16 ). Also, a trend in decreased platelet aggregation and basal thromboxane formation could be observed, but the most striking fact was a significant lowering of the diastolic blood pressure. In contrast, no differences could be observed in a placebo group receiving the same amount of sunflower oil, which of course contained a high proportion of linoleate and no n-3 PUFA( Reference Véricel, Calzada and Chapuy 16 ).
More recently, we have conducted another investigation with increasing intakes of pure DHA esterified in TAG (algal oil) in a population of middle-aged men (53–65 year-old) with each of them following the supplementation programme (2 weeks of successively 200, 400, 800 and 1600 mg DHA/d), making each volunteer his own control. A significant dose-dependent accumulation of DHA could be observed in platelet phospholipids, but platelet aggregation was only significantly lowered after the intermediate dosages (400 and 800 mg/d). In terms of redox status, only the 200 mg/d dosage was able to increase platelet vitamin E. Most interesting was to find that urinary isoprostanes, a recognised marker of oxidative stress, were significantly decreased after 200 mg/d and significantly increased after 1600 mg/d( Reference Guillot, Caillet and Laville 17 ). It must be noticed that other markers of oxidative stress taken into consideration in this study, were not significantly altered following the highest dosage. Regarding the plasma LDL, their phospholipids and cholesteryl esters dose-dependently accumulated DHA, but significant improvements of the redox status were observed after 200, 400 and 800 mg/d DHA intake only. This concerned vitamin E with the highest increase after 200 mg/d, and reciprocal U-shape curves for malondialdehyde, a global marker of oxidative stress, and the oxidisability of LDL to copper ions, with a decreased malondialdehyde and increased lag phase of oxidation in response to copper( Reference Calzada, Colas and Guillot 18 ). These results clearly indicate that low daily intake of DHA (lower than 1 g/d) allows expression of an ‘antioxidant’ profile based on several blood markers. This beneficial profile was no longer observed following the highest dosage, with even a global increased oxidative stress as stated earlier with urinary isoprostanes.
Overall, and although the two latter intervention studies in healthy human subjects have not been conducted on a long-term basis, they clearly indicate that the amount of long-chain n-3 PUFA intake is an issue that must be taken into consideration.
Oxygenated metabolism of DHA and biological effects
Contrary to EPA, that has the structural feature of arachidonic acid (ARA) with an additional cis/Z double bond at C17, DHA is not oxygenated into prostanoid-like products by COX although it may inhibit the enzymes by competition, especially against ARA( Reference Rao, Radha and White 19 ). DHA may however be hydroxylated into 13-hydroxylated derivative by COX-2 or into 17(R)-hydroxylated derivative if COX-2 is treated by aspirin, the latter derivative being a substrate of the neutrophil 5-LOX to produce resolvins D1 and D2( Reference Serhan, Hong and Gronert 20 ). This production is likely to be very low as the rate conversion of DHA into its 17(R)-hydroxylated derivative has been found to be <1–5 % of the rate conversion of ARA into PGH2 ( Reference Sharma, Dong and Yuan 21 ). In contrast, DHA is a fairly good substrate of LOX to produce various hydroxylated end-products after reduction of the hydroperoxide intermediates by glutathione peroxidase. They are 4- and 7-OH through 5-LOX, 11- and 14-OH through 12-/n-9-LOX and 17-OH through 15-/n-6-LOX( Reference Lagarde, Bernoud-Hubac and Calzada 22 ). The oxygenation of DHA through the latter LOX has been studied in detail for the production of protectin/neuroprotectin D1( Reference Ariel and Serhan 8 ). In this case, the biosynthetic route seems to mimic leukotriene B4 production by 5-LOX from ARA, then leading to 10(R),17(S)diOH-4Z,7Z,11E,13E,15Z,19Z−22 : 6( Reference Mukherjee, Marcheselli and Serhan 23 , Reference Marcheselli, Mukherjee and Arita 24 ).
A double 15-/n-6-LOX end-product can also be produced, which is a geometric and stereoisomer of protectin/neuroprotectin D1. It is 10(S),17(S)diOH-4Z,7Z,11E,13Z,15E,19Z−22 : 6 and has been named PDX( Reference Chen, Fenet and Michaud 25 ). As mono-hydroxylated derivatives have been shown to inhibit the thromboxane-induced aggregation of human blood platelets( Reference Croset, Sala and Folco 12 ), we have investigated the inhibition of that function by PDX and some isomers. In summary, PDX inhibits dose-dependently platelet aggregation induced by collagen, ARA and the stable thromboxane A2 mimetic U-46619 (a stable analogue of PGH2). The inhibition power of PDX towards the aggregation induced by collagen or ARA was the same, but the inhibition of the U-46619-induced aggregation was about half, suggesting that PDX did not affect the release of ARA from phospholipids in response to collagen but equally inhibits platelet COX-1 and thromboxane A2 response( Reference Chen, Véricel and Lagarde 26 ). The inhibition of COX-1 was confirmed by studying the oxygenation of radiolabelled ARA, which proved the specific inhibition of COX-1 as the 12-/n-9-LOX activity was not affected. Interestingly, a stereoisomer of PDX (10(R) instead of 10(S)), other double 15-/n-6-LOX end-products from ARA and 22 : 3n-6 were as inhibitory as PDX, providing they have the E,Z,E conjugated triene geometry. In contrast, isomers having an E,E,Z or E,E,E (all-trans) conjugated triene geometry were inactive. The E,Z,E conjugated trienes oxygenated PUFA have been collectively named poxytrins( Reference Chen, Véricel and Lagarde 26 ). It is worth noting that PD1, which has an E,E,Z conjugated triene motif, is described as an anti-inflammatory molecule but as a weak inhibitor of ADP-induced aggregation, without being further potentiated by aspirin treatment( Reference Dona, Fredman and Schwab 27 ).
More recently, we have extended our investigations regarding PDX activities. First, we found that it inhibits purified COX-1 and COX-2 with a slightly stronger inhibition of COX-2 (M Liu, T Boussetta, K Makni-Maalej, MÃ le Fay, F Driss, J El-Benna, M Lagarde and M Guichardant, unpublished results). Second, we addressed the possibility of inhibiting the reactive oxygen species generation in human neutrophils, as a previous work has shown that punicic acid, which exhibits a Z,E,Z-conjugated triene motif, inhibits NADPH oxidase-induced reactive oxygen species production( Reference Boussetta, Raad and Lettéron 28 ). Indeed, we found such an inhibition with a dose-dependent effect( Reference Liu, Chen and Véricel 29 ) (M Liu, T Boussetta, K Makni-Maalej, MÃ le Fay, F Driss, J El-Benna, M Lagarde and M Guichardant, unpublished results). However, whereas PDX is active against platelet aggregation and COX activities in the submicromolar range, the inhibition of reactive oxygen species generation requires micromolar concentrations. Leukotriene production from ARA being an important feature in neutrophils, we also tested the effect of PDX upon the formation of 5-LOX products in these cells. No inhibition was found while the endogenous COX-2 activity was inhibited at similar range concentrations as those inhibiting reactive oxygen species production (M Liu, T Boussetta, K Makni-Maalej, MÃ le Fay, F Driss, J El-Benna, M Lagarde and M Guichardant, unpublished results). This indicates that in addition to its potential for inhibiting platelet function, PDX may also exhibit some anti-inflammatory activity, likely through the inhibition of COX-2.
Conclusion
DHA is a nutrient with several beneficial effects in preventing atherothrombogenesis if it is consumed in moderate amounts. In this respect, several international recommendations agreeing with 0.5 g/d seem reasonable to avoid some possible side-effects in terms of oxidative stress. Several mechanisms could contribute to the beneficial effects. Among them, the inhibition of COX activities and the DHA-oxygenated products, mainly through LOX activities, may act by complementary effects such as inhibition of platelet aggregation and immune-competent cell function (Fig. 1). Altogether the atherothrombogenesis could be reduced. However, it remains to prove that enough oxygenated products are generated in situ to account for the effects observed in vitro.
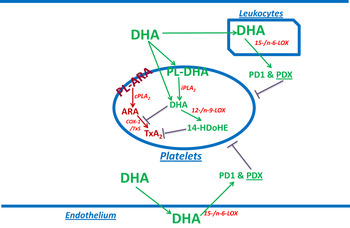
Fig. 1. (colour online) Summary of the DHA effect on blood platelets. DHA from the blood flow is partly taken up by platelets and mainly esterified into ethanolamine plasmalogens. This may be accompanied with an ‘antioxidant’ effect as shown by in vitro enrichment( Reference Véricel, Polette and Bacot 30 ). DHA may be released by calcium-independent phospholipase A2 (iPLA2)( Reference Strokin, Sergeeva and Reiser 31 ), and be converted into 14-hydroxy-docosahexaenoic acid (14-HDoHE) by 12-/n-9-LOX to inhibit thromboxane action. Some non-esterified DHA entering platelets may directly be converted into 14-HDoHE. Non-esterified DHA may also inhibit thromboxane A2 (TxA2) formation, in cPLA2-dependent activated platelets, through inhibition of COX-1. Besides platelets, DHA may be converted into protectin/neuroprotectin D1 (PD1) and protectin DX (PDX) by other cells containing 15-/n-6-LOX such as endothelial cells and leucocytes, and PDX may inhibit platelet aggregation. PL-ARA and PL-DHA, arachidonic acid and DHA-containing phospholipids, respectively. TxS, thromboxane synthase.
Acknowledgements
The authors thank the support of Inserm, the Ministry of Education and Research, ANR, and the Carnot LISA institute.
Financial Support
None.
Conflicts of Interest
None.
Authorship
M. L. wrote the review and supervised the experimental work. M. L., P. C. and F. D. conducted experiments. E. V., C. C. and M. G. conducted and supervised the experimental work.