Several epidemiological, intervention and animal studies point to a protective effect of a lignan-rich diet towards a range of lifestyle-related diseases such as certain cancers, CVD, and possible involvement in glycaemic control(Reference Adlercreutz1–Reference Bhathena and Velasquez3). Intervention studies with lignan-rich food such as flaxseed(Reference Jenkins, Kendall and Vidgen4) and rye bread(Reference Leinonen, Poutanen and Mykkanen5) reduce the cholesterol level in hypercholesterolaemic subjects. The high content of plant lignans in the diet may contribute to this hypocholesterolaemic effect. Increased enterolactone (ENL) concentrations in serum have been associated with a lower risk of CVD(Reference Vanharanta, Voutilainen and Rissanen6), and a high lignan intake to lower aortic stiffness(Reference van der Schouw, Pijpe and Lebrun7) and TAG level(Reference de Kleijn, van der Schouw and Wilson8). Mechanisms suggested to be involved in the protective effect of some lignans against CVD include antioxidative effects(Reference Kitts, Yuan and Wijewickreme9), stimulation of sex hormone-binding globulin synthesis(Reference Vanharanta, Voutilainen and Lakka10), up-regulation of LDL receptor activity(Reference Owen, Roach and Abbey11), inhibition of 7α-hydroxylase and acyl-CoA-cholesterol acyltransferase activity(Reference Sanghvi, Diven and Seltman12) and increased nitrogen oxide production(Reference Lee, Chen and Lin13).
Originally only secoisolariciresinol and matairesinol were identified as precursors of the enterolignans ENL and enterodiol (END). Improved methods of analysis led to the discovery of more precursors such as pinoresinol, syringaresinol, lariciresinol and medioresinol(Reference Heinonen, Nurmi and Liukkonen14, Reference Penalvo and Nurmi15), and recently further eighteen plant lignans were identified in food items including four found in rye and wheat(Reference Smeds, Eklund and Sjoholm16). These plant lignans account for 30–45 % of the total content of lignans in rye and 1–18 % of the total content in wheat(Reference Smeds, Eklund and Sjoholm16). The discovery of new enterolignan precursors and the recent development of methods to quantify them in food matrices, excreta and body fluids and tissues made possible the estimation of dietary lignan bioavailability and metabolism. We have recently reported that a diet based on wholegrain rye enriched with rye bran compared with wheat flour enriched with purified wheat fibre at iso-dietary fibre levels had a hypocholesterolaemic effect in hypercholesterolaemic pigs(Reference Lærke, Pedersen and Mortensen17). From the present study, it was concluded that the ability of dietary fibre from rye to interfere with digestion and absorption was more important for whole-body cholesterol homeostasis than regulation in the liver at gene level. Here we report new results on the quantitative metabolism and concentrations in biofluids and tissues of lignans in a pig model using high-fat cholesterol-enriched bread-based diets based on either wheat flour and refined wheat fibre, or wholegrain rye with added rye fibre.
Materials and methods
Diets
The study included two experimental diets: a high-fibre rye bread-based diet and a high-fibre wheat bread-based diet. Before administration of the high-fibre diets the pigs were fed an atherogenic diet for 2 weeks, followed by a wash-out period consisting of a low-fat wheat flour-based diet for 2–3 weeks as previously described(Reference Lærke, Pedersen and Mortensen17). The diets during the experimental period consisted of breads high in dietary fibre and enriched with fat and cholesterol (Table 1). The rye bread (produced at Holstebro Technical College, Denmark) contained finely ground wholegrain rye (Valsemøllen, Esbjerg, Denmark) and ground rye bran (Nordmills, Uppsala, Sweden), while the wheat bread (produced at Lantmännen Unibake, Karup, Denmark) contained white wheat flour supplemented with refined cellulose-rich wheat fibre (Vitacel WF 600; J. Rettenmaier & Söhne GmbH, Rosenberg, Germany) in order to achieve the same dietary fibre level. Whey protein concentrate (Lacprodan 87; Arla Foods Amba, Viby, Denmark) was used to adjust the protein content, and lard, egg powder (Danæg Products A/S, Roskilde, Denmark) and cholesterol (Sigma-Aldrich Co., St Louis, MO, USA) to increase the fat and cholesterol content. The diets were stored at − 20°C until consumption, and chromic oxide (Cr2O3) was added to ground experimental diets during the final 2·5 weeks of the study.
Table 1 Ingredients (g/100 g as-is)*, DM content (g/100 g as-is) and content of macronutrients and plant lignans (per 100 g DM) in the experimental breads
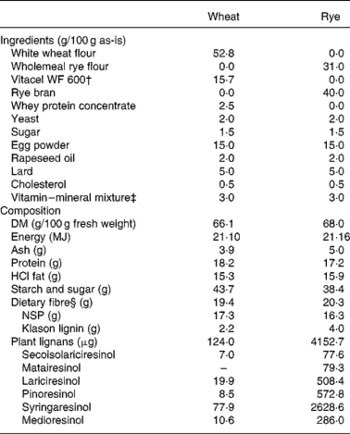
–, Not detectable.
* Before water addition and baking.
† J. Rettenmaier & Söhne GmbH (Rosenberg, Germany).
‡ Provided (mg/kg diet): Ca(H2PO4)2, 6642; NaCl, 4122; CaCO3, 16 580; FeSO4· 7H2O, 286; ZnO, 114; Mn3O4, 41; CuSO4·5H2O, 92; KI, 0·3; Na2SeO3·5H2O, 0·8; retinoacetate, 2·1; cholecalciferol, 0·03; α-tocopherol, 69; menadione, 2·52; riboflavin, 4·58; d-pantothenic acid, 12·59; cyanocobalamin (vitamin B12), 0·025; thiamin (vitamin B1), 2·52; niacin, 25·2; pyridoxine (vitamin B6), 3·78; biotin, 0·063.
§ Dietary fibre was calculated as the sum of NSP and Klason lignin.
Experimental design and animals
A total of seventeen Duroc × Danish Landrace × Yorkshire gilts, aged 4 months and obtained from Aarhus University, Faculty of Agricultural Sciences (Foulum, Denmark), were used for the study. The seventeen pigs, responding to ad libitum feeding of the atherogenic diet for 2 weeks by having elevated plasma cholesterol levels (7·2 (sem 0·51) mmol/l), were subsequently fed the low-fat wash-out diet for 2–3 weeks, by which the cholesterol level fell again to 2·5 (sem 0·08) mmol/l(Reference Lærke, Pedersen and Mortensen17). Following this, the pigs were fed the experimental diets for 6–7 weeks, followed by about 2·5 weeks where the breads were ground and Cr2O3 (2 g/kg on an as-is basis) was added. In week 2 after chromium addition to the diets the pigs were transferred to metabolism cages for 7 d, where faeces and urine were collected quantitatively on a daily basis and pooled per pig. Urine was collected over 4 g ascorbic acid per bottle, and pH of the collected urine was measured on a daily basis. The pigs were subsequently returned to their pens and euthanised 2–4 d after the end of the balance period. When not placed in metabolism cages, the pigs were housed individually in 4 m2 smooth-walled pens with a concrete floor. The feed intake during the period, where the pigs ate the experimental bread-based diets, was restricted to 2 kg/d increasing to 3 kg/d for about the last 2·5 weeks of the study.
Postprandial blood samples from the jugular vein, lateral auricular artery, hepatic vein and portal vein were taken into heparinised vacutainers 3 h after the morning meal from anaesthetised pigs. The pigs were subsequently euthanised with an overdose of sodium pentobarbital followed by exsanguination(Reference Lærke, Pedersen and Mortensen17). Samples were taken directly from the gall bladder and the urine bladder and stored frozen. Biopsies taken from the left lobe of the liver, tissue of the mid colon, breast and the brain were quick-frozen in liquid N2, and stored at − 80°C until analysis.
The animal experiment was conducted according to protocols approved by the Danish Animal Experiments Inspectorate and complied with the guidelines of the Danish Ministry and Justice concerning animal experimentation and care of animals under study.
Analytical methods
All analyses were performed in duplicate. Freeze-dried samples of diets and faeces were ground to a particle size of less than 0·5 mm before analysis. The DM content of feed and freeze-dried faeces was determined by drying to constant weight at 105°C for 20 h. Cr2O3 concentrations in faeces and diets were analysed as described by Schürch et al. (Reference Schürch, Lloyd and Crampton18). Macronutrient composition of the diets was analysed as previously described(Reference Lærke, Pedersen and Mortensen17).
A total of six plant lignans, namely secoisolariciresinol, matairesinol, lariciresinol, pinoresinol, medioresinol and syringaresinol, and two enterolignans, END and ENL, were profiled in the different samples. The content of plant lignans in the diets was analysed by isotope dilution GC-MS in the selected ion-monitoring mode (ID-GC-MS-SIM) as previously described(Reference Penalvo, Haajanen and Botting19). The same instrumental technique was used for the determination of plant lignans and enterolignans in faeces, using the corresponding extraction protocol(Reference Heinonen, Nurmi and Liukkonen14). The lignan profile in urine(Reference Nurmi, Voutilainen and Nyyssonen20), plasma and bile(Reference Penalvo, Nurmi and Haajanen21) was analysed by HPLC coupled with a coulometric electrode array detector (HPLC-CEAD).
The procedure for determination of lignans in tissues was as follows. Freeze-dried liver and brain tissue samples were ground to a fine powder, whereas colon and breast tissues were homogenised by crushing frozen with an Ultra Turrax T8 (IKA Werke GmbH & Co. KG, Staufen, Germany). To 50 mg of the samples and controls 300 μl distilled water were added and the samples were sonicated for 10 min. 3H-labelled oestradiol-17β-glucuronide (18 000 counts per min, NEN®; Perkin-Elmer, Shelton, CT, USA) in methanol (MeOH)–water (9:1, v/v) was added to serve as an internal standard for recovery correction. Thereafter 700 μl MeOH were added and the sample kept overnight at − 20°C in order to precipitate proteins and lipophilic material. The samples were centrifuged (3000 rpm) for 10 min below 0°C, and the supernatant fraction collected. The precipitate was washed once with 1 ml ice-cold ( − 20°C) 70 % MeOH, centrifuged. The two supernatant fractions were combined, and evaporated under N2 at 50°C until only water remained (500 μl), and subsequently still extracted twice with 2·5 ml n-hexane by vortex mixing for 4 min. The water phase was frozen in a dry-ice ethanol bath, and the n-hexane was discarded. The remaining traces of n-hexane were evaporated from the sample, and the water phase (500 μl) was hydrolysed by adding 500 μl of hydrolysis reagent containing β-glucuronidase (0·2 U/ml), sulfatase (2 U/ml) and 0·1 m-acetate buffer (pH 5·0) and incubated overnight at 37°C. The samples were extracted twice with 3 ml diethyl ether, mixing for 4 min, then evaporated to dryness and reconstituted in 500 μl MeOH. A 3 cm column of ion exchanger QAE-Ac in MeOH was prepared in a disposable Pasteur pipette and the sample in MeOH was applied to the column. The sample vial was rinsed once with 300 μl MeOH and applied to the column. The lignans were further eluted with 4 ml MeOH, and evaporated to dryness. For the HPLC analysis, the dry sample was reconstituted with 100 μl MeOH, 100 μl HPLC mobile phase (20 % phase B/A; see below) was added, and 10 μl immediately subjected to HPLC-CEAD. A subsample of 20 μl was taken for determination of [3H]oestradiol-17β-glucuronide recovery to correct for losses during the procedure. The mean recovery of [3H]oestradiol-17β-glucuronide for liver and colon tissues was 62·4 % (CV% 14·7) and for brain and breast tissues 84·2 % (CV% 10·4).
We used an HPLC system (ESA Biosciences, Inc., Chelmsford, MA, USA) equipped with a model 540 autosampler, two model 580 solvent pumps, and a model 5600 CEAD with eight electrode pairs. The analytical column was an Inertsil ODS-3 (GL Sciences Inc., Tokyo, Japan), 3 × 150 mm with particle size 3 μm, connected to a Quick Release RP-18 (Upchurch Scientific Inc., WA, USA) 3 × 10 mm guard column. The mobile phases used in HPLC-CEAD were: (A) 50 mm-sodium acetate buffer–MeOH (80:20, v/v); (B) 50 mm-sodium acetate buffer (pH 5·0)–MeOH–acetonitrile (40:40:20, by vol.). The chromatographic conditions were: detection potential, 660 mV; flow rate, 0·3 ml/min. The separation of compounds was carried out using gradient elution. All standards were dissolved in MeOH–20 % B/A (1:1, v/v). The seven-point standard curve ranged from 3·5 to 1393 ng/ml for ENL. No other lignans were detected in the tissue samples.
All lignan analyses included hydrolysis of any conjugate (glucosides in the diets, glucuronides and sulfates in the plasma and bile) and therefore the results are expressed as aglycones.
Calculations and statistical analysis
The apparent digestibility of the individual lignans (X) was calculated relative to the concentration of Cr2O3 as:

where X (d) and X (f) denote the concentrations of the lignans (X) in diet and faeces, respectively, and Cr 2O 3(d) and Cr 2O 3(f) are the corresponding concentrations of Cr2O3.
The estimated production of enterolignans (ELprod) was calculated as:

where PLintake, PLfaeces and PLurine are the quantitative intake and excretion of plant lignans in faeces and urine.
The excretion of enterolignans (ELexcretion) was calculated as:

where ELurine and ELfaeces are the sum of excreted ENL and END in faeces and urine during the 7 d collection period.
All statistical analyses were carried out using SAS for Windows (version 8.2; SAS Institute Inc., Cary, NC, USA). The level of significance was P < 0·05 in all analyses. Variables meeting the criteria of normal distribution are reported as least squares means (LSMeans) with their standard errors (sem). When variables were not normally distributed, statistical evaluation was performed on logarithmic transformations of the values, and results are reported as geometric means with 95 % CI.
The effect of diet and site of blood collection on plasma concentrations of lignans was examined by PROC MIXED, taking repeated measurements made on the same individuals into account(Reference Littell, Milliken and Stroup22).
The effect of diet on faecal and urine concentrations, digestibility, and excretion in urine and faeces were subjected to one-way analysis using PROC MIXED with GROUP option to account for variance heterogeneity.
Results
The markedly higher content of plant lignans in the rye diet compared with the wheat diet enriched with purified wheat dietary fibre (Table 1) resulted in a thirty-four times higher intake of lignans (Table 2). Syringaresinol was by far the dominating component of the rye diet, accounting for 60 % of the total plant lignan content, while secoisolariciresinol and matairesinol accounted for only 4–6 % of the total dietary intake of lignans.
Table 2 Intake, faecal excretion and digestibility of lignans from pigs fed the experimental wheat or rye diets
(Least squares means (LSMeans) with their standard errors)

–, Not detectable.
LSMean was significantly different from that for wheat: * P < 0·05, ** P < 0·01, *** P < 0·001.
† For digestibility calculation, see Calculations and statistical analysis section.
‡ Including isolariciresinol in faeces.
The higher intake of plant lignans with the rye diet was reflected in a twenty-eight times higher total faecal excretion of plant lignans and enterolignans compared with the wheat diet (Table 2). Enterolignans accounted for 34–57 % of the total faecal excretion of lignans, while 43–66 % was in the form of plant lignans.
The apparent faecal digestibility of the plant lignans was, in decreasing order: pinoresinol > medioresinol > secoisolariciresinol > syringaresinol > lariciresinol > matairesinol (Table 2).
As could be expected from the higher intake, there was a much higher concentration of enterolignans in plasma at all sites (portal vein, hepatic vein, lateral auricular artery and jugular vein) of pigs fed the rye compared with the wheat diet (Table 3). ENL was the primary circulating lignan accounting for 58–85 % of the total lignan content in plasma obtained from all four sites of collection. END, on the other hand, accounted for only approximately 1 % of the circulating lignans. Overall, the concentration of lignans was highest in the portal and hepatic vein, while the concentration was significantly reduced in peripheral venous and arterial blood.
Table 3 Concentration (nmol/l) of lignans in plasma collected 3 h postprandially from the portal vein, hepatic vein, a peripheral artery (lateral auricular artery) and jugular vein of pigs fed fibre-enriched wheat or rye diets*
(Geometric means and 95 % confidence intervals or least squares means (LSMeans) with their standard errors)

ENL, enterolactone; END, enterodiol; SECO, secoisolariciresinol; MAT, matairesinol; IsoLar, isolariciresinol; LAR, lariciresinol; PIN, pinoresinol; SYR, syringaresinol; MED, medioresinol; –, below detection limit.
* For portal vein, lateral auricular artery and jugular vein: n 8 for wheat and n 9 for rye. For hepatic vein: n 8 for wheat and n 7 for rye.
The bile of the wheat-fed pigs contained no measurable lignans, whereas the bile from the rye-fed pigs contained 6·2 (sem 0·9) μmol/l. Plant lignans accounted for 77 % of the measured lignans, and was dominated by matairesinol followed by lariciresinol, and secoisolariciresinol (Fig. 1). Enterolignans in the form of ENL accounted, on the other hand, only for 23 % of the total lignan content in bile from the rye-fed pigs.

Fig. 1 Concentration of lignans (μmol/l) in bile taken from the gall bladder 3 h postprandial of pigs fed fibre-enriched rye diets. Values are least squares means, with standard errors represented by vertical bars. ENL, enterolactone; END, enterodiol; SECO, secoisolariciresinol; MAT, matairesinol; LAR, lariciresinol; PIN, pinoresinol; SYR, syringaresinol; MED, medioresinol.
Both concentrations of lignans in bladder urine and in urine collected over a 7 d collection period were higher in pigs fed the rye compared with the wheat-fed pigs (Table 4). The lower concentration in urine from the 7 d collection compared with bladder urine may in part be explained by dilution with drinking water spillage collected from the cages together with urine. However, for calculation of quantitative excretion, this lower concentration is counteracted by the collection of larger volumes. Based on the 7 d quantitative collection of urine, and the concentration of lignans herein, the total urinary excretion of plant lignans and enterolignans was calculated to be 1·4 (sem 0·02) μmol/d for pigs fed the wheat diet, whereas the urinary excretion was 71·0 (sem 5·79) μmol/d for pigs on the rye diet (P < 0·0001). While plant lignans could not be detected in urine of the wheat-fed pigs, they contributed with 7·6 (sem 0·72) μmol/d for pigs on the rye diet.
Table 4 Concentration (nmol/l) of lignans in urine collected during a 7 d balance period or taken directly from the bladder at slaughter of pigs fed fibre-enriched wheat or rye diets
(Least squares means (LSMeans) with their standard errors)

LSMean was significantly different from that for wheat: * P < 0·05, ** P < 0·01, *** P < 0·001.
Significant concentrations of enterolignans in the form of ENL were found in the colon, liver and breast tissue, and minor concentrations in the brain of the rye-fed pigs (Table 5). ENL was also found in lower concentrations in the colon, liver and breast tissue of the wheat-fed pigs, while no measurable lignan was found in brain tissue. Thus, the highest concentrations are found in tissues that are in close proximity to the production site, the gut, and the organ for reconjugation, the liver.
Table 5 Concentration of enterolactone (pmol/g fresh weight) in various tissues of pigs fed fibre-enriched wheat or rye diets
(Least squares means (LSMeans) with their standard errors)

–, Below detection limit.
*** LSMean was significantly different from that for wheat (P < 0·001).
The estimated production of enterolignans calculated from the intake and excretion of plant lignans was 174 (sem 1·4) μmol/d for the rye compared with 5 (sem 0·1) μmol/d for the wheat diet (Fig. 2). Thus, the estimated production of enterolignans was 1·5–2·5 times higher than the 2 (sem 0·2) and 106 (sem 4·9) μmol/d actually recovered in faeces and urine from the wheat- and the rye-fed pigs, respectively.

Fig. 2 Estimated production (□) and calculated excretion () of enterolignans (μmol/d) of pigs fed fibre-enriched wheat or rye diets. Values are least squares means, with standard errors represented by vertical bars.
Discussion
The diets were formulated to be similar in macronutrients but different in dietary fibre characteristics and content of plant lignans(Reference Lærke, Pedersen and Mortensen17). As the plant lignans are concentrated in the aleurone and pericarp/testa layer of the rye kernel(Reference Nilsson, Aman and Harkonen23, Reference Glitso, Mazur and Adlercreutz24) the experimental rye bread enriched with rye bran had a lignan content that was about seven times higher than in ordinary rye bread (594 μg/100 g DM)(Reference Milder, Arts and van de Putte25). Lignan analysis of the diets confirmed other studies showing that secoisolariciresinol and matairesinol contribute only marginally to the total intake of plant lignans(Reference Penalvo, Haajanen and Botting19, Reference Milder, Arts and van de Putte25, Reference Hedelin, Klint and Chang26).
The proportion of the ingested plant lignans that were excreted in faeces in the form of enterolignans was lower in the present study than in a similar study with pigs fed wheat and rye diets (50–76 %)(Reference Bach Knudsen, Serena and Kjaer27). The main reason is that fewer lignans were identified previously, and only matairesinol and secoisolariciresinol were analysed in faecal material(Reference Bach Knudsen, Serena and Kjaer27). In the faeces of human subjects on a habitual diet, 34–35 % lignans were excreted as enterolignans(Reference Heinonen, Nurmi and Liukkonen14, Reference Adlercreutz, Fotsis and Lampe28, Reference Adlercreutz, Fotsis and Kurzer29). It has previously been shown that the amount of excreted lignans was higher in pigs than in human subjects, while the excretion per g ingested dietary fibre was identical(Reference Glitso, Mazur and Adlercreutz24).
The apparent digestibility of the individual plant lignans was generally higher than of the sum of plant lignans and enterolignans, reflecting that some lignans were converted to enterolignans before faecal excretion. The difference in digestibility among the plant lignans may partly be caused by conversion into intermediate plant lignans as previously shown in vitro (Reference Heinonen, Nurmi and Liukkonen14), and subsequent excretion without further conversion to enterolignans. The higher digestibility of lariciresinol, pinoresinol and syringaresinol in the present study than the estimated 10–19 % in a previous study(Reference Bach Knudsen, Serena and Kjaer27) is most probably a consequence of differences in the method of determination (direct or indirect), and a higher microbial activity due to a longer period of adaptation to the diet in the present study. Metabolites of fermentation of syringaresinol have been detected in vitro but not identified(Reference Heinonen, Nurmi and Liukkonen14). To what extent these metabolites, directly or indirectly, contribute to the faecal excretion of plant lignans in modified form is presently not known. However, based on the in vitro conversion their contribution to the excretion of enterolignans is considered to be low.
The increased levels of enterolignans at all sites of blood collection confirm several intervention studies(Reference Juntunen, Mazur and Liukkonen30–Reference McIntosh, Noakes and Royle33), cross-sectional(Reference Kilkkinen, Stumpf and Pietinen34–Reference Johnsen, Hausner and Olsen36) and animal studies(Reference Bach Knudsen, Serena and Kjaer27), where positive associations between intake of rye and wholegrain product and the ENL concentration in plasma or serum have been found. The higher concentrations of plant lignans and enterolignans in the portal vein than in the hepatic vein confirm previous findings that a substantial part of the lignans participate in the enterohepatic circulation or are converted to other metabolites. The significantly higher concentration of lignans in portal than in arterial blood is considered a consequence of the enrichment of the portal blood due to absorption from the gut(Reference Bach Knudsen, Serena and Kjaer27). Generally, enterolignans are considered to be absorbed more efficiently than plant lignans(Reference Niemeyer, Honig and Kulling37). Nevertheless, in the present study plant lignans accounted for 15–23 % of the total lignan concentration in plasma. Some enterolignans may be reconverted to plant lignans in the liver before excretion to the intestine by the biliary route(Reference Glitso, Mazur and Adlercreutz24) as indicated by the remethylation of the lignans arctiin and tracheloside in the liver of rats(Reference Nose, Fujimoto and Nishibe38). In vitro it has also been shown that ENL and END can undergo oxidative biotransformation by hepatic microsomes from rats and humans, which has been confirmed by the in vivo finding of several hydroxylated and methylated metabolites of ENL and END in the urine and bile of flaxseed-fed rats(Reference Niemeyer, Honig and Lange-Bohmer39).
In the present study, the very high proportion of plant lignans found in bile and a higher proportion in portal blood compared with the other blood vessels suggest that plant lignans are diverted toward systemic elimination via the bile. It has previously been shown that simple monobenzene derivatives with molecular weight below 300 kDa were poorly excreted in the bile, while compounds containing two or more rings and a highly polar anionic group with a minimum molecular weight of 325 kDa were effectively excreted(Reference Millburn, Smith and Williams40). The authors suggested that foreign compounds resembling the endogenous compounds (bile acids) would be effectively excreted in the bile.
Whether the excretion of the plant lignans by the biliary route is a protective mechanism only occurring after a systemic overload at very high intakes of plant lignans is not known. Because the plant lignan values are higher than the ENL values it seems that most of the ENL formed may pass the liver like oestriol-3-glucuronide for immediate excretion in urine(Reference Aubertin-Leheudre, Gorbach and Woods41), while the plant lignans may be selectively taken up by the liver cells, conjugated and excreted in the bile.
Before conjugation the plant lignans are certainly biologically active in the liver cells or in any other cell in the body. However, in conjugated form as in the bile, the lignans will not be biologically active again before they reach the intestine, are deconjugated by the microflora, and continue in a second enterohepatic circulation.
To what extent the plant lignans have any potential protective effect against diseases such as cancer and CVD if not converted to enterolignans is not yet fully elucidated. The conversions to END and ENL are not necessary steps for biological action, but the relative importance of plant lignans respective to enterolignans is currently not known. We know very little about the biological effects of plant lignans, but high intake of matairesinol has been related to lower breast cancer risk(Reference Adlercreutz1, Reference Piller, Verla-Tebit and Wang-Gohrke42). Different in vitro assays suggest that both plant lignans and enterolignans have antioxidative capacity(Reference Kitts, Yuan and Wijewickreme9, Reference Niemeyer and Metzler43–Reference Pool-Zobel, Adlercreutz and Glei45) with the antioxidative activities of secoisolariciresinol and matairesinol being significantly higher than that of ENL and END using a ferric-reducing/antioxidant power assay (FRAP)(Reference Niemeyer and Metzler43). The additional aromatic methoxy group in the plant lignans was suggested to be responsible for the higher antioxidant activities(Reference Niemeyer and Metzler43). However, the antioxidative effect has not been confirmed in human intervention studies(Reference Pool-Zobel, Adlercreutz and Glei45–Reference Hallund, Ravn-Haren and Bugel48).
Ingestion of foods rich in plant lignans leads to higher urinary ENL concentrations(Reference Nesbitt, Lam and Thompson49, Reference Hutchins, Martini and Olson50). The present results confirm previous results obtained after rye and wheat consumption both with pigs(Reference Bach Knudsen, Serena and Kjaer27) and human subjects(Reference Juntunen, Mazur and Liukkonen30). Enterolignans were the dominating components of the lignans in urine, but plant lignans accounted for 11 % of the lignans found in urine excreted from the rye-fed pigs. This suggests that approximately one-tenth of the lignans taken up from the intestine escapes microbial conversion and are absorbed directly as plant lignans, as also suggested in studies with rats(Reference Niemeyer, Honig and Kulling37, Reference Smeds, Saarinen and Hurmerinta51). A large variation in the pattern of plant lignans and enterolignans has been observed in the urine of human subjects(Reference Nurmi, Voutilainen and Nyyssonen20), where the excretion of plant lignans was higher than for those of enterolignans in two of ten subjects. Ingestion of secoisolariciresinol led to an increased excretion of lariciresinol in the urine of rats(Reference Smeds, Saarinen and Hurmerinta51), where lariciresinol was the main component of the excreted plant lignan also seen in the present study. This demonstrates that plant lignans can be converted to other plant lignans in vivo. The high excretion of lariciresinol may at least in part be caused by the high intake of pinoresinol as suggested by in vitro microbial conversion results obtained in a previous study(Reference Heinonen, Nurmi and Liukkonen14).
In the present study there was a slightly lower conversion from plant lignans to enterolignans than in the study of Bach Knudsen et al. (Reference Bach Knudsen, Serena and Kjaer27) that used comparable diets. In that study, the conversion was estimated to be 48 and 60 % for pigs on the wheat- and rye-based diets, respectively, and the enterolignans were primarily excreted via the faeces (46 % of ingested plant lignans). In the present study only 9 and 20 % of ingested plant lignans from the wheat and rye breads, respectively, were recovered as enterolignans in the faeces, while 27 and 35 % of ingested plant lignans were recovered in the urine as plant lignans or enterolignans. This is in the same range as previously observed in rats given radiolabelled secoisolariciresinol diglucoside(Reference Rickard and Thompson52), but slightly lower than in human studies with flaxseeds, where approximately 40 % of the ingested secoisolariciresinol diglucoside was excreted as enterolignans in the urine(Reference Kuijsten, Arts and Vree53). Based on the assumption that the colonic microflora would efficiently deconjugate the majority of lignans, only unconjugated lignans were determined in the faeces(Reference Glitso, Mazur and Adlercreutz24, Reference Adlercreutz, Fotsis and Kurzer54). If present at higher levels than anticipated, conjugated lignans may have contributed to the discrepancy between estimated production and measured excretion of enterolignans in the present study. They would also result in lower true faecal digestibility of the plant lignans than the calculated apparent digestibilities presented here. Another minor contribution may be caused by oxidation to other metabolites(Reference Niemeyer and Metzler43, Reference Jacobs, Kulling and Metzler55, Reference Smeds, Saarinen and Eklund56). Finally, quantitative losses of urine and/or further microbial activity in the collection bottle may contribute to the gap between estimated production and the actual recovery of enterolignans. The lower concentration of lignans in urine collected quantitatively than in the urine collected directly from the bladder is believed mainly to be caused by dilution with drinking water, but may also reflect losses as suggested above.
In contrast to the present study, previous studies have recovered two to ten times more enterolignan in faeces and urine than was expected from the intake of precursors(Reference Glitso, Mazur and Adlercreutz24, Reference Juntunen, Mazur and Liukkonen30), the main reason being the incomplete knowledge of the amounts of enterolignan precursors in the diet. Conversion of lignin to lignans is another possible contributor to enterolignan excretion both in the present and previous studies. Previously it was found that lignin accounted for 26–32 % of the ENL production in rats fed rye and wheat bran(Reference Begum, Nicolle and Mila57).
Body retention could potentially also contribute to the difference between estimated production and excretion, as enterolignans in the form of ENL were detected in various tissues. However, in the present study accumulation is expected only to parallel the growth of tissue, as a ‘steady-state’ condition can be expected after continuous ingestion for 9–10 weeks. Peroral exposure to 3H-labelled secoisolariciresinol diglycoside from flaxseed in rats has shown that the majority of radioactivity was recovered in the faeces and urine, while of twelve tissues analysed the highest concentrations were found in the liver, kidney and uterus(Reference Rickard and Thompson52). The high concentrations in these tissues after chronic exposure (10 d) was argued to be a consequence of the liver being the main site of conjugation with glucuronide, a high concentration of anti-oestrogenic binding sites in the liver, significant renal excretion of lignan metabolites, and the antagonising oestrogen action of enterolignans in the uterus(Reference Rickard and Thompson52). A study in rats fed diets enriched with linseed meal showed dose-related increases of END and ENL in the liver, testes, prostate and lung tissue(Reference Murray, Kang and Astheimer58). The present study is to our knowledge the first to demonstrate ENL in various tissues after intake of high amounts of rye. The moderate levels of ENL found in breast tissue and high levels found in plasma could definitely have biological activity. Previously it was shown that ENL accumulates in breast cysts, and that it modulates the risk related to high intracystic levels of epidermal growth factor(Reference Boccardo, Lunardi and Petti59), and it has recently been shown that higher blood concentrations of ENL reduce the risk of breast cancer. While Olsen et al. (Reference Olsen, Knudsen and Thomsen60) found an incidence rate ratio of 0·71 per 20 nmol/l higher plasma ENL associated with oestrogen receptor-α-negative tumours, Sonestedt et al. (Reference Sonestedt, Borgquist and Ericson61) showed that blood concentrations above 16 nmol/l diminished the risk for breast cancer in oestrogen receptor α-positive (OR 0·75) and oestrogen receptor β-negative (OR 0·60) tumours. Hence, ENL appears to modulate oestrogen receptor function, but the protective effect against breast cancer is probably mediated through several other mechanisms and factors related to the food consumed, particularly cereal fibre intake(Reference Adlercreutz1, Reference Suzuki, Rylander-Rudqvist and Ye62).
We have previously demonstrated an attenuating effect on plasma total and LDL-cholesterol of the diets in the hypercholesterolaemic pigs used in the present study. Lignans have been proposed to exert a cholesterol-lowering effect by stimulation of the LDL receptor activity(Reference Owen, Roach and Abbey11), although this could not be confirmed by the expression of mRNA in the liver of the pigs from the present study(Reference Lærke, Pedersen and Mortensen17). Currently, it is not known to what extent the lignans contribute to the hypocholesterolaemic effect obtained. The protective effect of whole grain against cancers and CVD as seen in some but not all epidemiological studies may be brought about by many mechanisms as discussed in a recent review(Reference Adlercreutz1). To what extent the lignans play an essential role by themselves, or are merely biomarkers, is still debated.
Conclusion
The present study shows that the lignans of rye are interconverted in the intestine and taken up to the systemic circulation to a variable extent. Some lignans are deposited in different tissues, but only ENL at a concentration high enough to be well quantified. At high intake levels, plant lignans are also taken up to the bloodstream and excreted via the urine and particularly via bile. This implies that the enterolignans and their precursors undergo enterohepatic circulation to a variable extent. The present study is to our knowledge the first to show that plant lignans are found in high concentrations in bile. Whether this is a mechanism of systemic elimination awaits further investigation.
Acknowledgements
H. N. L. was responsible for carrying out the animal experiment and data analysis. H. N. L. drafted the manuscript and is the corresponding author. M. A. M. and M. S. H. participated in conducting the animal experiment. K. E. B. K. was responsible for the project development and contributed with intellectual input to the planning, execution and evaluation of the experiment. J. L. P. and H. A. were responsible for developing and carrying out the lignan analyses. All authors contributed with intellectual input and writing of the manuscript.
Skilful assistance by the technical staff at University of Helsinki, Institute for Preventive Medicine, at Aarhus University, Department of Animal Health, Welfare and Nutrition and Department of Farm and Animal Research Facilities was greatly appreciated.
There are no conflicts of interests.