Introduction
A decline in woodland cover during the Early and/or Middle Roman period and subsequent woodland regeneration during the Late Roman and Early Medieval period, also called the Dark Ages, has been reported for many areas in northwestern Europe (e.g. Teunissen, Reference Teunissen1990; Dumayne & Barber, Reference Dumayne and Barber1994; Dark, Reference Dark1996, Reference Dark2000; Bunnik, Reference Bunnik1999; Wimble et al., Reference Wimble, Wells and Hodgkinson2000; Roymans & Gerritsen, Reference Roymans and Gerritsen2002; Becker, Reference Becker2005; Dreßler et al., Reference Dreßler, Selig, Dörfler, Adler, Schubert and Hübener2006; Groenewoudt et al., Reference Groenewoudt, van Haaster, van Beek and Brinkkemper2007; Kalis et al., Reference Kalis, Karg, Meurers-Balke and Teunissen-van Oorschot2008; Kaplan et al., Reference Kaplan, Krumhardt and Zimmermann2009; Ball & Jansen, Reference Ball and Jansen2018). The Roman period woodland decline is linked to population and economic growth and the creation of additional arable land and pasture, while the subsequent woodland recovery results from a decline in population and land use following the collapse of the western Roman Empire (Halsall, Reference Halsall2007; Cheyette, Reference Cheyette2008). In northern Belgium, woodland cover is believed to have been subject to the same evolution (Tack et al., Reference Tack, Van Den Bemt and Hermy1993; Verhulst, Reference Verhulst1995). As there are hardly any sedimentary archives that are suitable for pollen analyses, such as peat bogs or lakes, in this region covering the relevant period (Deforce, Reference Deforce2008; Storme et al., Reference Storme, Louwye, Crombé and Deforce2017), this reconstruction of the vegetation development is mainly based on indirect evidence. The few available pollen diagrams that cover the Late Roman and Early Medieval period have a low temporal resolution and show an increase in total arboreal pollen (AP) in only a small part of the diagrams and do not provide much detail on the evolution of individual taxa or on the timespan of these events (Verbruggen et al., Reference Verbruggen, Denys, Kiden, Berglund, Birks, Ralska-Jasiewiczowa and Wright1996; Broothaerts et al., Reference Broothaerts, Verstraeten, Kasse, Bohncke, Notebaert and Vandenberghe2014). Moreover, these sites are all located in alluvial landscapes where the recorded vegetational changes are likely to be influenced by dynamics of the local wetland vegetation, i.e. vegetational changes within the valley as a result of differences in local hydrology, rather than resulting from changes in land use on drier soils that are more suitable for agriculture.
This paper discusses changes in land use and related woodland dynamics during the Dark Ages based on the results of the analysis of botanical remains, including pollen, wood, charcoal and seeds, from a large-scale excavation of a rural Iron Age and Roman period agricultural settlement and the surrounding landscape near Kluizen (northern Belgium). During these excavations, several archaeological features, including wells and charcoal kilns, that represent small palaeoenvironmental archives spanning the Iron Age, Roman, Early and High Medieval period, have been found. The results of the analysis of the botanical remains from these features provide for the first time detailed information, from on-site sedimentary archives, on land use and vegetation dynamics during this period, and especially on the Late Roman and Early Medieval woodland regeneration following the abandonment of the settlement.
The results also provide new information on the drivers for the late Holocene expansion of Fagus in northern Belgium. In several regions in Europe, the late Holocene establishment and/or increase of Fagus has been studied extensively (e.g. Björkman, Reference Björkman1997; Tinner & Lotter, Reference Tinner and Lotter2006; Giesecke et al., Reference Giesecke, Hickler, Kunkel, Sykes and Bradshaw2007; Bradshaw et al., Reference Bradshaw, Kito and Giesecke2010). This paper now provides information on the the late Holocene dynamics of Fagus for a region where these dynamics are still poorly understood (Vandekerkhove et al., Reference Vandekerkhove, Deforce and Bastiaens2018).
Material and methods
Site – natural setting
The site Kluizen is located in northern Belgium (51°09′18.4″ N, 03°45′34.6″ E), 15 km north of the city of Ghent (Fig. 1). The region has an oceanic temperate climate with an annual precipitation of 833 mm evenly distributed throughout the year and a mean annual temperature of 10.4°C (KMI, 2018). It is situated on a small and low coversand ridge, at c. 5 m above mean sea level, with dry to wet sandy and loamy sand soils, and surrounded by lower-lying wet to very wet sandy and loamy sand soils. The potential natural vegetation in the area is alder carr (Alnion glutinosae) on waterlogged soil, beech-dominated (Fagion sylvaticae) or oak-hornbeam forest (Carpinion betuli) on dryer soils with high silt content, and English and sessile oak forest (Quercion robori-petraeaea) on sandy soils (Bohn et al., Reference Bohn, Neuhäusl, Gollub, Hettwer, Neuhäuslová, Raus, Schlüter and Weber2003; De Keersmaeker et al., Reference De Keersmaeker, Rogiers, Vandekerkhove, De Vos, Roelandt, Cornelis, De Schrijver, Onkelinx, Thomaes, Hermy and Verheyen2013).
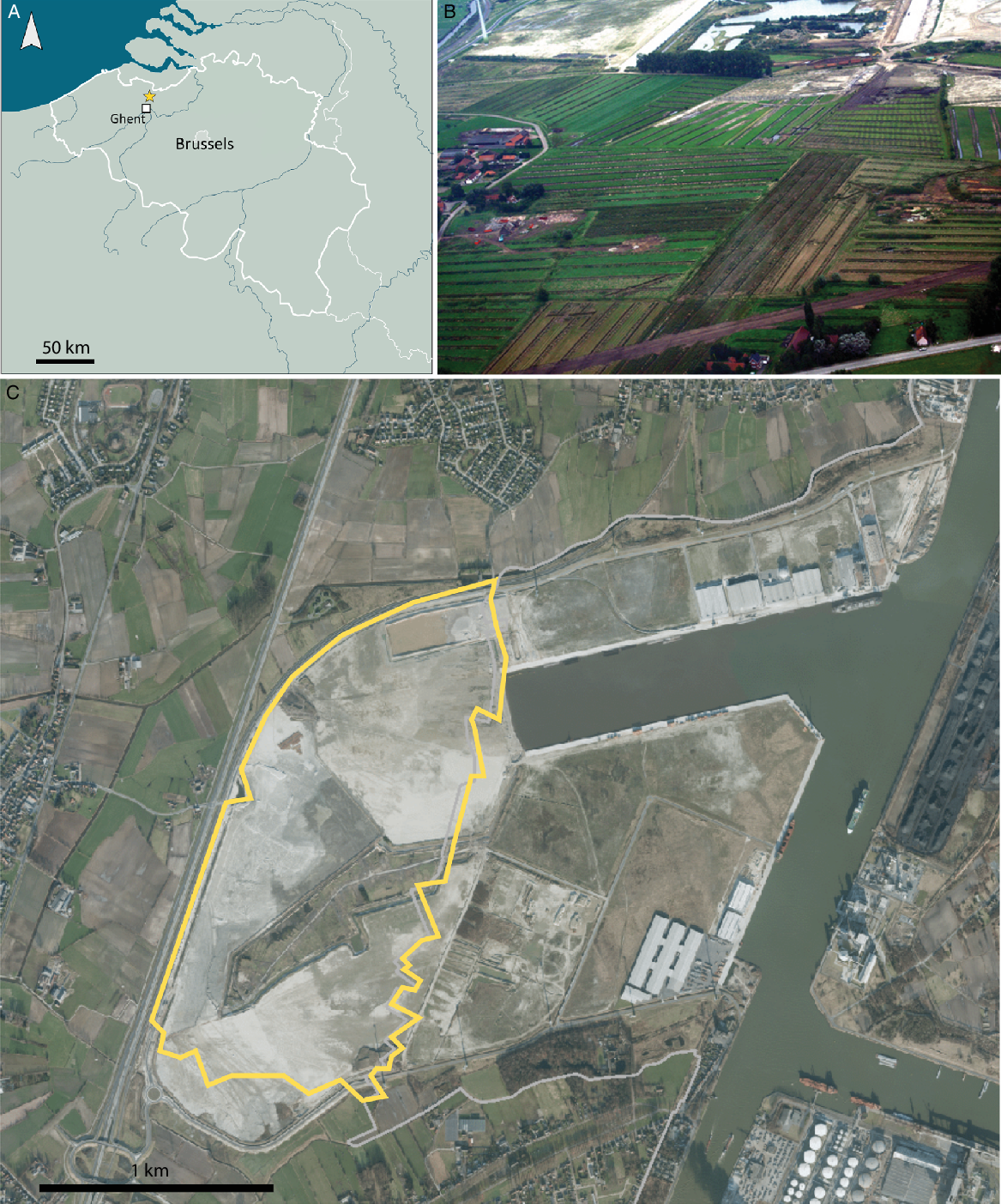
Fig. 1. Location of the study site (A); aerial view of the trial trenches (B) and of the studied area (perimeter indicated with yellow line) (C).
Site – archaeological and historical setting
During Roman times, the region to the north of Ghent was situated in the civitas Menapiorum, the most northern administrative part of Gallia Belgica, situated at the northwestern fringes of the Roman Empire. The studied site is located in the northern zone of the civitas: a region characterised by a strong persistence of native traditions, including continued use of timber house-building and the absence of towns and stone-built constructions such as villae. Notwithstanding this ostensible lack of Romanisation, the region witnessed a remarkable increase in rural occupation density from the Flavian period onwards (c. AD 70) and culminating in the mid-2nd century AD. From the late 2nd century onwards, settlement density rapidly declined and by the Late Roman period (c. AD 275–410) only a few settlements, probably of Germanic nature, are known, mostly located along the rivers and former Roman roads (De Clercq, Reference De Clercq2009, Reference De Clercq, Roymans and Derks2011).
From the Late Roman till the High Medieval period, the area north of Ghent seems to have been mostly deserted, with hardly any structures or finds known (Van Thienen, Reference Van Thienen2016; Fig. 2). During the 5th century, the Western Roman Empire crumbled and was replaced by the Frankish dynasty of the Merovingians, who in turn were succeeded by the Carolingians around the middle of the 8th century. During the disintegration of the Carolingian empire in the 9th century, the counts of Flanders emerge as the new local power. Possibly the first count of this dynasty, Baldwin I, already possessed the Ghent area in AD 864 (Dhondt, Reference Dhondt1942).

Fig. 2. Locations of known archaeological sites near the study region for each discussed time period. Timespan of cultural periods according to Slechten (Reference Slechten2004).
Historical documents describe the region as almost completely abandoned during the Early Middle Ages, with large forests and wastinae (heathland) covering the region between Bruges, Ghent and the river Scheldt (Verhulst, Reference Verhulst1965, Reference Verhulst1995). This is confirmed by the lack of archaeological data, with only one known Early Medieval site north of Ghent, situated c.3 km to the northwest of Kluizendok (S. Scheltjens & T. Apers, unpublished report, 2018). Other Early Medieval settlements are located c.12 km further south, respectively at Merendree (De Clercq, Reference De Clercq1997; De Logi & Van Cauwenbergh Reference De Logi and Van Cauwenbergh2010; De Logi, Reference De Logi2015) and Ghent (De Groote & Berkers, Reference De Groote, Berkers, De Groote and Ervynck2017; Vermeiren et al., Reference Vermeiren, Bru and Steurbaut2017). During the later 9th and 10th century, Ghent would develop into an important pre-urban trading centre (Verhulst Reference Verhulst1999; Vermeiren et al., Reference Vermeiren, Bru and Steurbaut2017).
During the High Medieval Period, the area to the north of Ghent was subject to intensified land conversion (Verhulst, Reference Verhulst1965, Reference Verhulst1995). This corresponds to the archaeological dataset, in which a noticeable increase in settlements occurs (Fig. 2d). An increase in rural settlement to the north of Ghent occurs in the early 11th century, with a maximum in the 12th century (11 excavations yielded settlement structures dating to this interval). In the same period, between 1115 and 1140, the village of Kluizen was founded as an exploitation settlement by the count of Flanders (Verhulst, Reference Verhulst1991).
Excavation, sampling and analysis
Between 2005 and 2009, a large-scale archaeological trial-trenching campaign over 160 ha was carried out prior to construction of a new dock in the harbour area of Ghent. During this evaluation, several clusters of mainly Roman-period archaeological features were recorded such as postholes, ditches and wells. A large number of charcoal (pit) kilns were also found. Based on the results of this first campaign, it was decided to excavate 16 ha area-wide. Until today, this is the largest contiguous area excavated in Belgium (Laloo et al., Reference Laloo, De Clercq, Perdaen, Crombé, Ampe, Bastiaens, Boeren, Boudin, Deforce, Degryse, Ervynck, Haneca, Langohr, Lentacker, Van Calster, Van den Berghe, Van Strydonck and Sergant2009a).
All studied archaeological structures were sectioned during excavation. The exposed profiles of the fills of wells and ditches were sampled for pollen analysis using monolith tins. Subsequently, 5 L bulk samples were taken for the analysis of botanical macro remains (seeds and charcoal) from different levels from these fills.
Subsamples for pollen analysis (c.1 cm3) were taken from the monolith tins in the lab and processed following standard procedures (Moore et al., Reference Moore, Webb and Collinson1991). The identification of pollen and spores was based on Moore et al. (Reference Moore, Webb and Collinson1991), Beug (Reference Beug2004) and a reference collection of modern pollen and spores. Percentages of the different pollen types are based on a pollensum (ΣP) of minimum 300 pollen grains, including all terrestrial plants.
Bulk samples for the analysis of seeds and fruits and charcoal (5 L sample size) were sieved using tap water, the smallest mesh being 0.5 mm. Seeds were picked until the whole sample or a representative subsample had been analysed. Identifications of seeds and fruits are based on the reference collection of Flanders Heritage Agency, Cappers et al. (Reference Cappers, Bekker and Jans2006), Anderberg et al. (Reference Anderberg1994) and Berggren (Reference Berggren1969, Reference Berggren1981).
For wood species identification transverse, radial and tangential thin sections were cut with a razor blade, mounted in glycerol (50%) and studied using a transmitted-light microscope (100–400×). Charcoal fragments were manually broken along transverse, radial and tangential planes and these surfaces were studied using an incident-light microscope (50–500×), using dark-field illumination. Identifications of wood and charcoal are based on Schweingruber (Reference Schweingruber1990) and Schoch et al. (Reference Schoch, Heller, Schweingruber and Kienast2004).
Ages of the archaeological structures were established using radiocarbon dating, dendrochronology and typological analysis of ceramics found in these structures. For all wells with a lining of poles and/or planks, the construction date was determined by tree-ring analysis of the individual wooden elements, of which the end dates were combined into a single felling date (range) for each feature. For some wells, the start and the end of the accumulation of sediments in their fill was radiocarbon-dated. The lower part of the fill was dated using botanical macro remains. The upper part of the wells was radiocarbon-dated using pollen-rich residues (Vandergoes & Prior, Reference Vandergoes and Prior2003) as these sediments did not contain any botanical macro remains.
Results
Archaeological features
Most of the 405 recorded archaeological features are ditches that bordered the farms, sand-tracks, postholes, charcoal (pit) kilns, cremation graves, (refuse) pits and water wells. Except for one Neolithic pit, and two wells and one pit dating to the Iron Age, the vast majority of these features are the remains of a Roman-period rural settlement that evolved from one enclosed farmstead built in the late 1st century AD into a cluster of three or more contemporaneous enclosed farms situated along a SW–NE-oriented unpaved road during the 2nd century AD (Fig. 3). As often observed in the northern part of civitas Menapiorum, the occupation subsequently shrunk during the 3rd century AD, with only one farm surviving. No archaeological features indicating habitation dating to the Late Roman or Early Medieval period were detected (Fig. 4). Human presence at the sites only resumed by the 9th or probably even the 10th century AD and was restricted to charcoal production. A detailed description of all excavated archaeological features is given by Laloo et al. (Reference Laloo, De Clercq, Perdaen, Crombé, Ampe, Bastiaens, Boeren, Boudin, Deforce, Degryse, Ervynck, Haneca, Langohr, Lentacker, Van Calster, Van den Berghe, Van Strydonck and Sergant2009a).

Fig. 3. Number and age of the different types of excavated archaeological features at the Kluizendok site (see Laloo et al. (Reference Laloo, De Clercq, Perdaen, Crombé, Ampe, Bastiaens, Boeren, Boudin, Deforce, Degryse, Ervynck, Haneca, Langohr, Lentacker, Van Calster, Van den Berghe, Van Strydonck and Sergant2009a,b) for details on the individual features). Only 15 charcoal kilns have been radiocarbon-dated. The other kilns have been classified Roman (rectangular kilns) or medieval (round kilns) based on their typology (cf. Groenewoudt, Reference Groenewoudt2007; Deforce et al., Reference Deforce, Vanmontfort and Vandekerkhove2018). The kilns classified as Roman are therefore likely to include Early Medieval kilns as well.

Fig. 4. OxCal multiplot summarising all calibrated radiocarbon (2σ ranges) and tree-ring dates, together with the date range of cultural finds (ceramics). All dendro-dates are to be interpreted as terminus post quem, except for R4/H Sp. 1019 which represents a felling date in AD 253.
Radiocarbon dates
In total, 42 samples were radiocarbon-dated (Table 1; Fig. 4) and converted to calendar dates with the IntCal13 atmospheric calibration curve (Reimer et al., Reference Reimer, Bard, Bayliss, Beck, Blackwell, Ramsey, Buck, Cheng, Edwards, Friedrich, Grootes, Guilderson, Haflidason, Hajdas, Hatté, Heaton, Hoffmann, Hogg, Hughen, Kaiser, Kromer, Manning, Niu, Reimer, Richards, Scott, Southon, Staff, Turney and van der Plicht2013) in OxCal v4.3.2 (Bronk Ramsey, Reference Bronk Ramsey2017). The oldest archaeological features date to the early Iron Age (2475 ± 30 BP) and coincide with the earliest possible date of the ceramics and with the dendrochronological date of a well lining (well WP15; see next section). Most radiocarbon dates from archaeological features related to habitation of the site, such as wells, postholes and cremation graves, are clustered in the first to fourth century AD. Bayesian modelling (Amodel = 90.2) indicates occupation most likely started between AD 22 and 88 and ended between AD 291 and 358 (68.2% probability). This is a slightly wider age range compared to the age obtained from the typological study of the ceramics which indicates that the occupation of the site ceased around AD 270, which can be explained by the course of the radiocarbon calibration curve around this period.
Table 1. Radiocarbon dates from the Kluizendok excavation. Calibration is done with OxCal v4.3.2 (Bronk Ramsey, Reference Bronk Ramsey2017) using the IntCal13 calibration curve (Reimer et al., Reference Reimer, Bard, Bayliss, Beck, Blackwell, Ramsey, Buck, Cheng, Edwards, Friedrich, Grootes, Guilderson, Haflidason, Hajdas, Hatté, Heaton, Hoffmann, Hogg, Hughen, Kaiser, Kromer, Manning, Niu, Reimer, Richards, Scott, Southon, Staff, Turney and van der Plicht2013).

The radiocarbon dates of the charcoal kilns cluster in three age groups. The oldest group (n = 7) is contemporaneous with the archaeological features related to the rural Roman-period settlement. The second group (n = 5) dates to the end of the Early Medieval period or the early High Medieval period (c. AD 800 to 1000) and the third group (n = 3) dates to the middle or late High Medieval period (c.1000 – 1200 AD).
The ages obtained from the fill of the wells indicate that these deposits have accumulated over a very long period after the wells went out of use. Though the accumulation of sediment in the wells was not necessarily continuous, the radiocarbon dates indicate that it covered the Late Roman and Early Medieval period. In the Iron Age well (WP15), this period was even longer, from the Late Iron Age till the High Medieval period.
Dendrochronological dates
A total of 110 wooden elements from the linings of 12 water wells were sampled for dendrochronological analysis. After careful selection, the tree-ring pattern of 94 samples (all Quercus sp.) was recorded using a Lintab™ measuring table, internally synchronised with TSAPWin (Rinn Reference Rinn2003) software and cross-dated against absolutely dated local site and master chronologies from Flanders and surrounding regions (Table 2).
Table 2. Dendrochronological dates from the Kluizendok excavation.

The measured tree-ring series display a high variability in terms of their length (number of tree rings on a cross section), the presence of abrupt growth variations and average ring-width. All measured series were internally synchronised, within and between features, and when multiple series displayed a high visual and statistical correlation (based on t BP values (Baillie & Pilcher, Reference Baillie and Pilcher1973) and the percentage of parallel variation (Eckstein & Bauch Reference Eckstein and Bauch1969)), a chronology was computed. Those chronologies were then cross-dated against a recently composed tree-ring chronology of Iron Age timbers from the Netherlands and northern Belgium (S. van Daalen & K. Haneca, unpublished data) and local chronologies of dated Roman timbers from northern Belgium (Haneca, Reference Haneca2009; Laloo, Reference Laloo, De Clercq, Perdaen, Crombé, Ampe, Bastiaens, Boeren, Boudin, Deforce, Degryse, Ervynck, Haneca, Langohr, Lentacker, Van Calster, Van den Berghe, Van Strydonck and Sergant2009a).
Wood from two water wells could be attributed to the Iron Age. The lining of one of these (WP11), dated after 167 BC, was clearly built with reused planks from an older construction. This well also contained Roman-period ceramics at the base of its fill and is therefore considered to be linked to the Roman habitation. From well WP15, two wooden elements have an end date in 615 and 628 BC. The tree-ring series dated to 615 BC includes 11 sapwood rings, which allows the felling date for this timber to be located between 614 and 589 BC. This is supported by a radiocarbon date on a piece of roundwood (with bark attached) from the same lining (KIA-36468: 2415 ± 25 BP) that has a calibrated date range between 731 and 404 BC. Three timbers used for the lining of this well were identified as elements originating from an ard plough (Laloo et al., Reference Laloo, De Clercq, Crombé, Deforce, Haneca and Perdaen2009b), of which one is dendro-dated (end date 628 BC). These exceptional wooden finds were added to WOODAN: an online database of wooden archaeological finds (Haneca et al., Reference Haneca, Deforce, Lange, van der Laan and Nicolaij2019) (www.woodan.org: IDs 21184000, 21185000 and 21186000).
Six wells could be dated within the Roman period, based on intercomparison and cross-dating against absolutely dated chronologies (Haneca, Reference Haneca2009) (Table 1). The presence of sapwood or waney edge allowed a narrow time window to be determined for the felling date of trees used for the well linings. For three other wells only a terminus post quem could be reported as no sapwood was present on any of the dated series from these well linings.
Seeds and fruits
Seeds and fruits have been studied from the fill of wells (n = 20), storage pits (n = 2), refuse pits (n = 10), charcoal kilns (n = 7), cremation graves (n = 6), ditches (n = 10) and sunken byres (n = 2). Uncharred remains dominate the samples from the wells, while in the other features charred remains are present almost exclusively. (Tables with both summary data for the wells (cultivated plants, trees and shrubs; Table SM1) and full data for all features (Tables SM2 and SM3) are given in the Supplementary Material available online at https://doi.org/10.1017/njg.2020.11.)
Despite the high number of studied samples, only a limited number of remains from cultivated plants have been found, i.e. some cereals, Panicum miliaceum being present almost throughout (Iron Age and Roman period), and some scarce finds of oil plants (Camelina sativa subsp. sativa, Linum usitatissimum), nuts (Juglans regia), kitchen herbs (Coriandrum sativum) and pulses (Vicia faba var. minor). In contrast, shrubs and trees are very well represented, with at least 20 taxa, showing a high ecological diversity, from wet to dry and from light-demanding to shade-tolerant. They occur in 16 wells out of 20, with the highest number of taxa in the Iron Age well WP15 (n = 10) and the Roman well WP12 (n = 11).
Wild herbaceous taxa are best represented by species from grasslands, pioneer vegetation (including arable land) and woodlands in the Iron Age and Roman wells, and only a few species from tall herb vegetation and aquatic vegetation have been found. The representation of trees and shrubs is remarkable, as woodland species are generally underrepresented in the archaeobotanical record in comparison to modern flora (Cappers, Reference Cappers1994). Therefore it is assumed that trees and shrubs were growing close to the wells and/or recolonised the site quickly after abandonment (secondary succession).
Wood identification
A total of 422 pieces of wood from the lining of the excavated wells have been studied (Table 3). The lining of the Iron Age well (WP15) was a circular construction of pointed stakes made from Alnus sp., Betula sp., Corylus avellana, Fraxinus excelsior, Quercus sp. and Salix sp. The Roman wells were either circular and lined with wickerwork, or had a rectangular to square outline and were lined with a construction made of beams and planks, many of which showed traces indicating these have been reused from other wooden constructions. Wickerwork linings were made with vertical stakes made from Corylus avellana, Salix sp., Fraxinus excelsior, Prunus sp., Alnus sp. and Quercus sp. and horizontal twined twigs of mostly Salix sp., although some twigs of Corylus avellana, Quercus and Alnus sp. were also used. Rectangular to square lined constructions were made from Quercus sp., Alnus sp., Betula sp. and Fraxinus excelsior (Table 3).
Table 3. Wood identifications from well linings from the Kluizendok excavation.

Charcoal analysis
Charcoal has been studied from six Roman-period domestic refuse deposits, six Roman cremation graves and from ten Roman and five Early or High Medieval charcoal kilns, resulting in a total of 2753 analysed charcoal fragments (Table SM4 in the Supplementary Material available online at https://doi.org/10.1017/njg.2020.11).
Charcoal assemblages of the domestic refuse deposits are dominated by Alnus sp. (51.9%). Quercus sp. (13.6%) and Corylus avellana (7.8%) are relatively important as well. Other taxa that have been found include Acer sp., Betula sp., Fagus sylvatica, Fraxinus excelsior, Maloideae, Prunus sp., Salix sp. and Ulmus sp., but all in low percentages.
The charcoal assemblages of the cremation graves are dominated by Alnus sp. (four graves) or Quercus sp. (two graves). Betula sp. is also abundant in two of the studied graves. Very small amounts of Corylus avellana, Fagus sylvatica and Ilex aquifolium have been identified as well. The charcoal assemblages of the graves are further characterised by a very low taxonomic diversity, with between one and three different taxa identified from each of the graves.
Except for two Roman-age charcoal kilns where Fagus sylvatica is also important, all studied charcoal kilns are dominated by Quercus sp. Only very small amounts of Alnus sp., Betula sp., Carpinus betulus, Clematis vitalba, Frangula alnus, Fraxinus excelsior, Salix sp. and Sambucus sp. have been identified.
Pollen analysis
Pollen analysis has been done on the fill of the two Iron Age wells and several of the Roman-period wells (11), ditches (8) and pits (2) that have been excavated.
The pollen diagrams of several of the wells and ditches show a similar evolution of the vegetation (Fig. 5). The lower part of the sandy fill of the well shows little evolution in the percentages of the individual taxa. AP percentages generally fluctuate between 40% and 60% (but sometimes up to 80%) in the Roman-period wells and ditches, and between 75% and 93% for the Iron Age wells, and are mostly composed of wetland trees (basically Alnus) and early successional trees (Betula, Corylus and Salix). In the upper part of the sandy fill, pioneer tree taxa decrease and middle successional trees, mostly Quercus, increase. The part of the pollen diagrams corresponding to the peaty infill of the wells at first shows a further increase of Quercus, which later decreases and is replaced by later successional trees such as Fagus, Carpinus and Ilex. Finally, in the upper part of most of the diagrams, late successional trees decrease and percentages of pioneer taxa rise again.

Fig. 5. Summary pollen diagrams for Iron Age well WP15 (A) and Roman-period wells WP1 (B) and WP12 (C). Complete pollen diagrams for all studied features are given in Laloo et al. (Reference Laloo, De Clercq, Perdaen, Crombé, Ampe, Bastiaens, Boeren, Boudin, Deforce, Degryse, Ervynck, Haneca, Langohr, Lentacker, Van Calster, Van den Berghe, Van Strydonck and Sergant2009a). Successional status of trees and shrubs according to Finegan (Reference Finegan1984), Leuschner & Ellenberg (Reference Leuschner and Ellenberg2017) and Peterken & Lloyd (Reference Peterken and Lloyd1967). Dates are the calibrated age probability distributions (2σ range) or dendro-dates (TPQ: terminus post quem).
Taphonomy of the wells
Most of the above-described palaeoenvironmental data originate from the fill of different wells. These are small, on-site and man-made sedimentary basins and the accumulation of botanical material in these structures might be influenced by anthropogenic activities and discontinuous sedimentation (van Amerongen, Reference van Amerongen2020). The low numbers of ceramics, other artefacts, charcoal and other charred plant remains, especially the extremely low percentages of cultivated plants, absence of animal bones etc. in the fill of the wells, are clear indications that no refuse has been thrown in the wells, however, and that most of the botanical remains reflect the local vegetation, rather than resulting from human activities (Fig. 6A). Also, a well is regularly cleaned when in use, since a well largely filled with sediment is of no use to the inhabitants of the site (Greig, Reference Greig1988; van Amerongen, Reference van Amerongen2020). Therefore, most of the infill of these wells is considered to have accumulated after the abandonment of the structure (Greig, Reference Greig1988; Vanhoutte et al., Reference Vanhoutte, Bastiaens, De Clercq, Deforce, Ervynck, Fret, Haneca, Lentacker, Stieperaere, Van Neer, Cosyns, Degryse, Dhaeze, Dijkman, Lyne, Rogers, van Driel-Murray, van Heesch and Wild2009).

Fig. 6. Cross section of the lower part (A) and upper part (B) of the fill of one of the wells. Scale bar = 1 m.
The data also indicate that the accumulation of sediment in these wells was discontinuous or happened at a very irregular speed. Both the lower content of organic material and the evolution in the pollen diagrams, with early successional trees being dominant for most of this part of the fill, indicate that the initial sandy infill of the wells accumulated shortly after abandonment. Another argument for the rather fast sediment accumulation in the lower part of the structures is that these were filled in before the wooden linings could collapse.
The upper peaty layer (Fig. 6B) spans a much longer period, as shown by the radiocarbon dates, i.e. most of the Early Medieval and the start of the High Medieval period. The abrupt changes in the sedimentology and in the pollen diagrams indicate, however, that there might have been a period without or with very slow sediment accumulation between these two sedimentary units. Why the sedimentation resumed and/or changed remains unclear. The accumulation of peaty sediment might be a result of a change in local hydrology/soil humidity as a consequence of a decrease in temperature and increase of precipitation during the Early Medieval period (Büntgen et al., Reference Büntgen, Tegel, Nicolussi, McCormick, Frank, Trouet, Kaplan, Herzig, Heussner, Wanner, Luterbacher and Esper2011, Reference Büntgen, Myglan, Ljungqvist, McCormick, Di Cosmo, Sigl, Jungclaus, Wagner, Krusic, Esper, Kaplan, de Vaan, Luterbacher, Wacker, Tegel and Kirdyanov2016; Helama et al., Reference Helama, Jones and Briffa2017). Also the establishment of a dense forest cover at the site, resulting in a higher accumulation rate compared to the decomposition of organic material in the small depressions, might have contributed to the accumulation of the peaty sediment.
Because of their small catchment size, the pollen assemblages from the fill of these wells, or from the small remaining depressions when the wells were filled for the most part, are not reliable sources of information on the regional vegetation (Sugita, Reference Sugita1994; Sugita et al., Reference Sugita, Gaillard and Broström1999; van Amerongen, Reference van Amerongen2020) but will mainly reflect the vegetation from the immediate surroundings of these features (Jacobson & Bradshaw, Reference Jacobson and Bradshaw1981). In fact, the wells or remaining depressions can be considered as very small forest hollows, which will capture the pollen deposition from the vegetation growing only 20–50 m away (Jacobson & Bradshaw, Reference Jacobson and Bradshaw1981; Bradshaw, Reference Bradshaw1988; Calcote, Reference Calcote1995; Jackson & Kearsley, Reference Jackson and Kearsley1998). As a result, the pollen diagrams from Kluizen must reflect the changes in the vegetation on a stand-scale, i.e. on the (deserted) site itself and the former cultivated plots directly bordering the site.
Land use and vegetation evolution
Prehistoric and proto-historic period
A very extensive area has been excavated, but this resulted in only some scarce archaeological features and (lithic) finds predating the Roman period. This indicates that the area was probably not, or just ephemerally, exploited during the Stone Age (Mesolithic and Neolithic) and Bronze Age. Initial occupation started during the Iron Age and was rather limited in size. One well dating to the Early Iron Age and one dating to the Late Iron Age have been found at the study site, indicating a small-scale settlement in the vicinity, though other Iron Age structures pointing towards habitation have not been found. No continuity in human habitation from the Iron Age into the Early Roman period could be observed. The ard fragments used in the construction of well WP15 indicate agricultural activities during the Early Iron Age, and also remains of cultivated plants such as Panicum miliaceum and Camelina sativa potentially demonstrate the presence of local crop fields. Most taxa of the macro remains in the fill of this well, however, are trees and shrubs (Table SM1 in the Supplementary Material available online at https://doi.org/10.1017/njg.2020.11), including some typical old-growth forest species such as Ilex aquifolium and Taxus baccata. Together with the pollen assemblages from the lowermost part of the fill, with AP percentages of over 75% (Fig. 5A), this indicates a woodland-dominated landscape, with probably only small-scale clearings. Higher up in the fill of the well, pioneer tree taxa show an important increase, with first Salix and then Corylus, indicating woodland recovery after abandonment of the Early Iron Age habitation. Towards the base of the peaty layer in this well, which has been dated to the Late Iron Age (382–204 BC), these pioneer taxa are replaced by Quercus. This is followed by a short decrease in AP probably corresponding to the Roman-period settlement.
Roman period
The excavated archaeological features indicate that a small rural settlement was established at the site from c. AD 70 onwards, with probably not more than three farms in use at the same time, and which was abandoned by c. AD 270. The farmers seem to have applied a mixed regime of agriculture and animal husbandry, the latter being testified by the presence of enclosures and stable parts of the houses. The few remains of cultivated plants suggest local production of cereals, but as these are mainly from Panicum miliaceum, soil conditions might have been too poor for the cultivation of most other cereal crops.
In the lower part of the fill of the Roman features, AP vary between c.40% and c.60%, indicating a lower woodland cover compared to the Early Iron Age landscape at the site. AP-percentages and thus presumably forest cover were higher compared to most other Roman-period sites in northern Belgium (e.g. Cooremans et al., Reference Cooremans, Desender, Ervynck and Schelvis2002; Deforce, Reference Deforce2012; Vanhoutte et al., Reference Vanhoutte, Dhaeze, Deforce, Demeyer, Ervynck, Haneca, Lentacker and Stieperaere2016) and the Netherlands (e.g. Groenewoudt et al., Reference Groenewoudt, van Haaster, van Beek and Brinkkemper2007), where AP values from wells and small pools generally vary between 15% and 40%. Also the macrobotanical assemblages from the Roman wells from Kluizendok point towards a wooded landscape, with a large number of different tree and shrub taxa in the assemblages of the lowermost part of their fill (Table SM1 in the Supplementary Material available online at https://doi.org/10.1017/njg.2020.11). Finally, the presence of several remains of Roman-period charcoal kilns is also a strong indication for the local presence of woodland (Groenewoudt & Spek, Reference Groenewoudt and Spek2016).
AP percentages increase in all of the investigated Roman features from the bottom of their fill onwards, reflecting woodland regeneration after abandonment of the site (Fig. 5). Initially, percentages of early successional trees such as Salix, Corylus and Betula show a significant rise, followed by increasing percentages of Quercus.
Early Middle Ages
The region has been abandoned since the Late Roman period and continued to be uninhabited during the Early Middle Ages. The only archaeological features that potentially date to this period and which indicate human activities are charcoal kilns (see next section, ‘Woodland exploitation’), with a radiocarbon age range between c. AD 800 and 1000 (Fig. 4; Table 1), but no settlement features, nor botanical remains from cultivated plants dating to this period have been found.
All peaty layers in the upper part of the fill of the Roman wells have been radiocarbon-dated in the Early Medieval period (Table 1; Fig. 4). The peaty layer in the fill of the Early Iron Age well WP15, however, seems to have started to accumulate already in the Late Iron Age. Most likely, the peaty layers in the upper part of the fill of the other Roman wells and ditches – which have not been radiocarbon-dated – also have an Early Medieval age. The similarity in sediment type, stratigraphic position and synchronous evolution of the different taxa in the corresponding parts of the pollen diagrams substantiate this assumption. Even the upper part of the peaty layer in the Iron Age well WP15 displays the same properties. All pollen diagrams display very high AP percentages of c.90% or higher, indicating a completely forested landscape (Fig. 5; Laloo et al., Reference Laloo, De Clercq, Perdaen, Crombé, Ampe, Bastiaens, Boeren, Boudin, Deforce, Degryse, Ervynck, Haneca, Langohr, Lentacker, Van Calster, Van den Berghe, Van Strydonck and Sergant2009a). First, Quercus shows a maximum in all diagrams, which is followed by an increase of late successional trees such as Fagus sylvatica, Ilex aquifolium and Carpinus betulus.
High Middle Ages
No archaeological features dating to the High Middle Ages that indicate habitation have been found during the excavations. The only features pointing towards human activities are charcoal kilns. In the corresponding part of the pollen diagrams, i.e. the top of the peaty layer in some of the wells (Fig. 5A, B), total AP percentages and late successional (and shade-tolerant) trees decline, indicating a more open woodland, which is confirmed by the increase of several light-demanding taxa such as Betula, Corylus and Salix. This is likely to be a consequence of felling of trees for charcoal production and potentially other human activities such as cattle-herding in the forest. At the end of the High Medieval period, between AD 1115 and 1140, the village of Kluizen was founded as an exploitation settlement by the count of Flanders (Verhulst, Reference Verhulst1991), which will have resulted in the final deforestation of the area.
The above-described vegetation evolution is even clearer from the composite pollen diagram using only the pollen assemblages of those levels of the fills that have been dated individually (Fig. 7). This diagram shows that AP percentages decrease during the Roman period, indicating a decline in woodland cover, which must be related to the conversion of forests and woodlands into arable land and pastures, as it is synchronous with an increase in archaeological features, both within the excavated area (Fig. 3) and in the wider region (Fig. 2). During the Early Medieval period, there is an important increase in AP, up to over 90, indicating an almost total woodland recovery in the study area. During the High Medieval period, AP percentages drop again.

Fig. 7. Composite pollen diagram showing pollen percentages of total arboreal pollen (AP) and of a selection of taxa from individually dated levels of the fill of different wells from the study site during the Iron Age (IA), Roman (ROM), Early Medieval (EM) and High Medieval (HM) period.
The Roman-period woodland clearance does not seem to have affected much of the woodland on wet soil, as Alnus percentages remain fairly stable (Fig. 7). Quercus does show an important decrease, indicating that preferentially the drier parts of the landscape were cleared for agriculture. Fagus, which was only a minor woodland element during the Iron Age and the Roman period, becomes a prominent tree species from c. AD 600 onwards. As the Roman settlement was completely abandoned by AD 270, and had already shrunk well before that date, the evolution from abandoned agriculture land, over pioneer forest and secondary (Quercus-dominated) forest to finally a forest with Fagus and other late successional trees such as Carpinus and Ilex took more than 300 years. This is a remarkably long period, as it is assumed that in the temperate zone of northwestern Europe, pioneer tree species are replaced by later successional trees within a timespan of about 100 years (Ellenberg, Reference Ellenberg1978; Packham & Harding, Reference Packham and Harding1982).
Woodland exploitation
For the Iron Age, little information is available on woodland exploitation, with only 17 wood fragments identified from the lining of a well and no charcoal from archaeological features. Both the selection of taxa and the construction of the lining of the well are similar to many other Iron Age wells from northern Belgium. These are generally simple circular constructions of pointed stakes and/or wickerwork constructions using a broad range of woody taxa (e.g. Cherretté Reference Cherretté2000; Deforce Reference Deforce2004; De Clercq et al., Reference De Clercq, Cherretté, De Mulder, Van Rechem, In’t Ven and De Clercq2005; Deforce & Minsaer Reference Deforce and Minsaer2005).
Far more data are available for the Roman period. Except for wickerwork, where Salix was the preferred wood type, Alnus and Quercus were preferentially used, both as construction wood for the wells, and as fuel for both domestic use and cremation rituals. The taxonomic diversity of the charcoal assemblages from the cremation graves is far lower compared to the refuse deposits, however, which is also characteristic of other Roman-period sites in Belgium and likely to be a result of different fuel selection strategies (De Groote et al., Reference De Groote, Bastiaens, De Clercq, Deforce and Vandenbruaene2003; Deforce & Haneca Reference Deforce and Haneca2012; Cerezo-Román et al., Reference Cerezo-Román, Henrotay, Deforce, Van Neer, Cerezo-Román, Wessman and Williams2017). A low taxonomic diversity is also characteristic of the charcoal kilns, which are strongly dominated by Quercus, and which has also been observed in previous studies of Roman and Early Medieval kilns from Belgium (Deforce et al., Reference Deforce, Marinova and Dalle2015, Reference Deforce, De Clercq, Hoorne, Laloo, Boudin, Vanstrydonck and Crombé2017, Reference Deforce, Vanmontfort and Vandekerkhove2018). The youngest Roman-period kilns show higher percentages for Fagus sylvatica, compared to the older Roman kilns. This indicates that the increase of Fagus – as observed in the pollen diagrams for the Early Medieval period – probably started already during the Roman period. The late Early Medieval or early High Medieval kilns are all dominated by Quercus and have no or only very small amounts of Fagus charcoal, though the pollen data show that Fagus must have been an important element in the local vegetation during the Early Medieval period. Both trees produce excellent charcoal, and F. sylvatica has been found to be dominant in Early Medieval charcoal kilns in central Belgium, which makes selection in favour of Quercus for charcoal production unlikely (Deforce et al., Reference Deforce, Vanmontfort and Vandekerkhove2018). Potentially, these kilns are all younger than the Early Medieval Fagus maximum in the pollen diagrams, however, as the radiocarbon dates of these kilns all have a very wide age probability distribution.
Discussion and conclusions
The results from this study are concordant with numerous other investigations from northwestern Europe, where a phase of woodland regeneration is observed at the end of the Roman period and during the Early Middle Ages. For northern Belgium, however, information on the evolution of the vegetation during this period was largely missing and vague up to now. This study partially fills this gap as it documents the recolonisation by woodland vegetation of a Roman rural settlement following its abandonment. This woodland regeneration could not only be reconstructed on a stand-scale level, but also with a higher chronological resolution than previous studies from northern Belgium, showing the different successional stages of this spontaneous reforestation.
As the data in this study mostly reflects the local vegetation, it remains unclear how representative the observed vegetation evolution is for other regions in Belgium, however. Most studies that do provide palaeoenvironmental information for this period indicate some increase of woodland following the Roman period, for the coastal area (Deforce & Ervynck, Reference Deforce, Ervynck, Hillewaert and Ryckaert2019), the Scheldt valley (Verbruggen et al., Reference Verbruggen, Denys, Kiden, Berglund, Birks, Ralska-Jasiewiczowa and Wright1996; Storme & Deforce, Reference Storme and Deforce2011) and Central Belgium (Desender et al., Reference Desender, Ervynck and Tack1999; Van Impe et al., Reference Van Impe, In’t Ven, De Paepe, Ervynck and Desender2005; Broothaerts et al., Reference Broothaerts, Verstraeten, Kasse, Bohncke, Notebaert and Vandenberghe2014). But at least at some of these sites, (Early Medieval) habitation returned much faster following abandonment since the Late Roman period, and woodland succession was halted at an earlier stage, before late successional trees could become an important woodland component (Storme & Deforce, Reference Storme and Deforce2011; Deforce & Ervynck, Reference Deforce, Ervynck, Hillewaert and Ryckaert2019).
This study also provides new information on the role of Fagus sylvatica in the postglacial vegetation evolution of northern Belgium. Although Fagus was present in the vegetation since c. 4500 BP, and became the dominant tree in the woodland vegetation in central Belgium since c.2000 BP, it was believed never to have been an important element in the woodland vegetation of northern Belgium (Verbruggen et al., Reference Verbruggen, Denys, Kiden, Berglund, Birks, Ralska-Jasiewiczowa and Wright1996; Vandekerkhove et al., Reference Vandekerkhove, Deforce and Bastiaens2018). The data from Kluizen show that, at least in certain areas where settlements were abandoned for a long timespan following the Roman period, Fagus did become an important element in the woodland vegetation, with pollen percentages of 20–30%, after a long succession of the woodland vegetation. An important increase of Fagus during the late Holocene has been observed in several other regions of northwestern, northern and central Europe and is mostly explained by Fagus being promoted by human disturbance of the vegetation, especially along its northern range limit (Küster, Reference Küster1997; Bradshaw et al., Reference Bradshaw, Kito and Giesecke2010; Pędziszewska & Latałowa, Reference Pędziszewska and Latałowa2016). At many sites, this sudden increase of Fagus can be dated between AD 300 and 800 (e.g. Küster, Reference Küster1997; Bunnik, Reference Bunnik1999; Dreßler et al., Reference Dreßler, Selig, Dörfler, Adler, Schubert and Hübener2006), a period characterised by a cooler and moister climate (Büntgen et al., Reference Büntgen, Tegel, Nicolussi, McCormick, Frank, Trouet, Kaplan, Herzig, Heussner, Wanner, Luterbacher and Esper2011, Reference Büntgen, Myglan, Ljungqvist, McCormick, Di Cosmo, Sigl, Jungclaus, Wagner, Krusic, Esper, Kaplan, de Vaan, Luterbacher, Wacker, Tegel and Kirdyanov2016; Helama et al., Reference Helama, Jones and Briffa2017). In northern Belgium and other regions near its southwestern distribution limit, the sudden Fagus expansion is more likely to be explained by this change in climatic conditions, rather than anthropogenic disturbance, as it colonises the abandoned settlement only after a long succession of more than 300 years. Soil degradation, i.e. soil acidification and the leaching of nutrients following Iron Age and Roman-period forest clearings, might have contributed to this late expansion of beech in the observed forest succession. Given the small scale, relatively short period and probably low intensity of the Iron Age and Roman-period agricultural activities in this area, this is not likely to have played a major role, however.
Finally, this paper also adds to the growing number of studies that demonstrate the value of pollen analysis of on-site archaeological features for the reconstruction of local vegetation dynamics (e.g. Pokorný et al., Reference Pokorný, Boenke, Chytráček, Nováková, Sádlo, Veselý, Kunes and Jankovská2006; Groenewoudt et al., Reference Groenewoudt, van Haaster, van Beek and Brinkkemper2007; Halvorsen & Hjelle, Reference Halvorsen and Hjelle2017; Innes & Haselgrove, Reference Innes and Haselgrove2019). Especially in regions with no natural deposits such as peat bogs or lakes, which are traditionally used as sedimentary archives for vegetation reconstructions, these can be an alternative. Due to their small diameter and on-site location, archaeological features provide information on the vegetation on the site itself and its immediate surroundings, which is generally not possible with natural sedimentary archives from wetlands.
Acknowledgements
The administration for Maritime Access of the Flemish government is acknowledged for financing the archaeological fieldwork and the different analyses, and the NWO-funded Dark Ages project for financing this publication. Jelle Van den Berghe is acknowledged for help with the pollen analysis. The authors are also grateful for the constructive remarks made by the anonymous reviewers on earlier versions of the manuscript.
Supplementary material
To view supplementary material for this article, please visit https://doi.org/10.1017/njg.2020.11