97 results
14 - Algae in Extreme and Unusual Environments
- from Part II - Physiology of Photosynthetic Autotrophs in Present-Day Environments
-
-
- Book:
- Evolutionary Physiology of Algae and Aquatic Plants
- Published online:
- 24 October 2024
- Print publication:
- 07 November 2024, pp 272-292
-
- Chapter
- Export citation
Range extension for Conopea saotomensis (Crustacea; Cirripedia: Archaeobalanidae) in the tropical eastern Atlantic
-
- Journal:
- Journal of the Marine Biological Association of the United Kingdom / Volume 104 / 2024
- Published online by Cambridge University Press:
- 20 September 2024, e81
-
- Article
-
- You have access
- HTML
- Export citation
Chapter 14 - Facilitation
- from Part IV - Community Ecology
-
- Book:
- Ecology in Action
- Published online:
- 04 April 2024
- Print publication:
- 04 July 2024, pp 354-375
-
- Chapter
- Export citation
Chloroidium phycobionts (Watanabeales, Trebouxiophyceae) partner with lecanoralean mycobionts in foliicolous lichen communities of Tenerife (Canary Islands) and Navarra (Iberian Peninsula), Spain
-
- Journal:
- The Lichenologist / Volume 56 / Issue 2-3 / May 2024
- Published online by Cambridge University Press:
- 26 March 2024, pp. 107-119
- Print publication:
- May 2024
-
- Article
-
- You have access
- Open access
- HTML
- Export citation
Punctelia borreri and P. subrudecta (Parmeliaceae) associate with a partially overlapping pool of Trebouxia gelatinosa lineages
-
- Journal:
- The Lichenologist / Volume 55 / Issue 5 / September 2023
- Published online by Cambridge University Press:
- 22 September 2023, pp. 389-399
- Print publication:
- September 2023
-
- Article
-
- You have access
- Open access
- HTML
- Export citation
8 - Culture and Cooperation
-
- Book:
- Understanding Living Systems
- Published online:
- 22 June 2023
- Print publication:
- 06 July 2023, pp 113-130
-
- Chapter
- Export citation
1 - The Gene Delusion
-
- Book:
- Understanding Living Systems
- Published online:
- 22 June 2023
- Print publication:
- 06 July 2023, pp 1-13
-
- Chapter
-
- You have access
- HTML
- Export citation
6 - Learning from the Wood Mouse
-
- Book:
- Understanding Living Systems
- Published online:
- 22 June 2023
- Print publication:
- 06 July 2023, pp 83-98
-
- Chapter
- Export citation
9 - People of the Forest
-
- Book:
- Understanding Living Systems
- Published online:
- 22 June 2023
- Print publication:
- 06 July 2023, pp 131-146
-
- Chapter
- Export citation
7 - Artificial Intelligence
-
- Book:
- Understanding Living Systems
- Published online:
- 22 June 2023
- Print publication:
- 06 July 2023, pp 99-112
-
- Chapter
- Export citation
3 - What Evolves?
-
- Book:
- Understanding Living Systems
- Published online:
- 22 June 2023
- Print publication:
- 06 July 2023, pp 30-46
-
- Chapter
- Export citation
2 - Replication, Reproduction and Variation
-
- Book:
- Understanding Living Systems
- Published online:
- 22 June 2023
- Print publication:
- 06 July 2023, pp 14-29
-
- Chapter
- Export citation
4 - Purpose in Life
-
- Book:
- Understanding Living Systems
- Published online:
- 22 June 2023
- Print publication:
- 06 July 2023, pp 47-68
-
- Chapter
- Export citation
5 - Cry of the Wolf
-
- Book:
- Understanding Living Systems
- Published online:
- 22 June 2023
- Print publication:
- 06 July 2023, pp 69-82
-
- Chapter
- Export citation
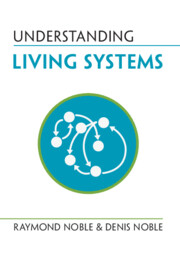
Understanding Living Systems
-
- Published online:
- 22 June 2023
- Print publication:
- 06 July 2023
8 - Our Microbiota and How to Do Gene Therapy in the Kitchen
- from Part I - Foundations: What Do We Need to Know about Optimal Aging?
-
- Book:
- Unaging
- Published online:
- 15 September 2022
- Print publication:
- 20 October 2022, pp 126-147
-
- Chapter
- Export citation
Evolutionary genomic insights into cyanobacterial symbioses in plants
-
- Journal:
- Quantitative Plant Biology / Volume 3 / 2022
- Published online by Cambridge University Press:
- 08 August 2022, e16
-
- Article
-
- You have access
- Open access
- HTML
- Export citation
3 - Rooted Life
- from Part II - Turning to Fundamentals
-
- Book:
- This Sacred Life
- Published online:
- 08 October 2021
- Print publication:
- 14 October 2021, pp 63-89
-
- Chapter
- Export citation
Complex photobiont diversity in the marine lichen Lichina pygmaea
-
- Journal:
- Journal of the Marine Biological Association of the United Kingdom / Volume 101 / Issue 4 / June 2021
- Published online by Cambridge University Press:
- 22 September 2021, pp. 667-674
-
- Article
-
- You have access
- Open access
- HTML
- Export citation
6 - Model-Theoretic Aspects
-
- Book:
- Gödel, Tarski and the Lure of Natural Language
- Published online:
- 03 December 2020
- Print publication:
- 17 December 2020, pp 134-158
-
- Chapter
- Export citation